Magdalena M. Schimke, Sabrina Marozin, and Günter Lepperdinger
Abstract
Multipotential mesenchymal stromal cells (MSC) are present as a rare subpopulation within any type of stroma in the body of higher animals. Prominently, MSC have been recognized to reside in perivascular locations, supposedly maintaining blood vessel integrity. During tissue damage and injury, MSC/pericytes become activated, evade from their perivascular niche and are thus assumed to support wound healing and tissue regeneration. In vitro MSC exhibit demonstrated capabilities to differentiate into a wide variety of tissue cell types. Hence, many MSC-based therapeutic approaches have been performed to address bone, cartilage, or heart regeneration. Furthermore, prominent studies showed efficacy of ex vivo expanded MSC to countervail graft-vs.-host-disease. Therefore, additional fields of application are presently conceived, in which MSC-based therapies potentially unfold beneficial effects, such as amelioration of non-healing conditions after tendon or spinal cord injury, as well as neuropathies. Working along these lines, MSC-based scientific research has been forged ahead to prominently occupy the clinical stage. Aging is to a great deal stochastic by nature bringing forth changes in an individual fashion. Yet, is aging of stem cells or/and their corresponding niche considered a determining factor for outcome and success of clinical therapies?
Keywords: vascular niche, cell-based therapy, aging biology, cellular dysfunction, age-associated pathology, regenerative medicine
Background
Mesenchymal stem cells (MSC) are multipotential precursor cells that maintain and repair tissues in the body of adult individuals. MSC have been isolated from embryonic tissues as well as from adult up to advanced ages (Landgraf et al., 2011; Batsali et al., 2013; Beane et al., 2014a; Todeschi et al., 2015). Yet experimental knowledge about in vivo activities in regenerating models is still scarce (Wang et al., 2013a; Zhao et al., 2015). Besides replenishing mesenchymal tissues, MSC also modulate haematopoiesis as well as immune response (Pontikoglou et al., 2011; Hao et al., 2012; Law and Chaudhuri, 2013; Bianco, 2014). Conceivably residing in perivascular locations, MSC are identified with cells better known as pericytes. This cell type is involved in maintaining blood vessel integrity under normal conditions. During tissue damage and injury, MSC are thought to become instantaneously activated and by evading from their perivascular niche to support wound healing and tissue regeneration (Murray et al., 2014; Wong et al., 2015).
MSC are acknowledged for their potential to regenerate damaged tissue due to their ability to terminally differentiate into a broad variety of cell types. Deliberately, stem cells are perceived being ageless by nature. Yet, it is by now generally accepted that, with advancing age, a decline of stem cell function and activity has its share in delaying the replacement and the turnover of damaged cells in compromised renewable tissues (Bajek et al., 2012; Bethel et al., 2013). Also, stem cells in their niches are exposed to threads such as reactive oxygen species, harmful chemical agents or physical stresses, which trigger premature senescence, provoke accelerated cell death or cellular transformation (Li et al., 2014a). In osseous tissues at an advanced age, both mass and mineral density of cortical and cancellous bone steadily decreases. At the same time, fat cells emerge within the bone marrow and muscles. Fat cell-specific expedition of systemically deteriorating adipokines and pro-inflammatory cytokines primes the emergence of age-associated diseases. Hence, aged or senescent circumstances call for advanced therapies (Reitinger et al., 2015). Scientific approaches aiming at standardized medical treatment often neglect these biological and patho-physiological constraints. Nevertheless, these should be distinctly considered. Otherwise rightly conceived and diligently established strategies are bound to fail.
Unresolved questions regarding phenotypic appearance and in vitro techniques
Biological properties
Stromal cell types exhibit characteristic features. The rather large spindle-shaped cells present microvilli on their surface and produce extracellular matrix, which together facilitates MSC to firmly adhere to cell culture plastic (Friedenstein, 1976; Castro-Malaspina et al., 1980). This property is often exploited to isolate and culture-purify MSC from biopsies (Owen and Friedenstein, 1988). Variant culture conditions significantly impact on cell adhesion and consequently isolation outcome and MSC expansion. Therefore, inconsistencies often arise when employing inappropriate brands of cell culture plastic and media supplements.
MSC immunophenotype
Another selection criterion for MSC is a tri-lineage differentiation potential forming osseous, adipose, and cartilaginous progenitors (Mark et al., 2013; Patrikoski et al., 2014), and a distinguished immune phenotype positive for CD105, CD73, and CD90, and negative for CD45, CD34, HLA-DR, and other markers (Dominici et al., 2006; Al-Nbaheen et al., 2013). This marker canon is not always unequivocal, as other cell types may also fulfill these criteria. MSC-like cells often exhibit differential marker expression depending on tissue origin and period of culture expansion (Gronthos et al., 1999; Wagner et al., 2005; Kaiser et al., 2007; Riekstina et al., 2008). A prominent example is the surface marker STRO-1. Due to the availability of a highly affine monoclonal antibody, STRO-1 has not only gained popularity as a marker but also for use in cell enrichment (Stewart et al., 1999). Endothelial cells may however also express STRO-1 thus questioning the specificity of this marker (Lin et al., 2011; Ning et al., 2011). Though, the likely equivalence of MSC to vascular pericytes reconciles STRO-1 being a good marker for true MSC (Feng et al., 2011; Chen et al., 2012; da Silva Meirelles et al., 2015). Another currently debated marker is CD34. Previously, MSC were considered CD34 negative, yet adipose-derived MSC express CD34 (Lin et al., 2008; Baer, 2014). Likewise, CD271 and CD146 markers have also been described (Rasini et al., 2013; Busser et al., 2015; Cuthbert et al., 2015). During culture expansion marker expression can change. Whether, these changes reflect the biological age of MSC has not been thoroughly studied. Also specific markers amenable for the quantification of MSC age are so far not available. In appreciation of this experience, further standards in MSC validation are needed, in particular when surface antigens expression differs in primary vs. culture-expanded MSC. Together with the restricting biological constraint of biological and replicative age, also tissue origin has to be accounted.
Isolation techniques
To enhance isolation yields, often cell purification and fractionation by density gradient centrifugation is performed. This procedure bears the enhanced risk of contamination and therefore requests skilled operators. To reduce variability, closed, semi-automated separation devices, granting higher recovery rates, have been engineered. Their operational speed and efficiency warrant reproducible processing thereby, easing standardization for fulfilling compliance criteria in “Good Manufacturers Practice” (GMP) (Ito et al., 2010; Otsuru et al., 2013, 2015). Production of clinical-grade MSC must be performed in accordance to GMP standards and compels not only reproducibility, but also scalability. Working along the same line, novel automated cell platforms have been introduced for production of higher cell numbers in reduced times and passages (Roberts et al., 2012; Nold et al., 2013; Rojewski et al., 2013; Hanley et al., 2014). Gaining a pure MSC culture is difficult in particular when attempting to erase single-lineage committed progenitors or contaminating hematopoietic cells (Kerk et al., 1985; Kuznetsov et al., 1997). Therefore, selection enrichment using fluorescence-activated cell sorting (FACS) or magnetic-activated cell sorting (MACS) has been introduced. Although FACS was initially favored because it provides higher purities, shear stress within the fluids compromised cell viability, and chemotaxis (Deschaseaux et al., 2003; Rada et al., 2011; Li et al., 2013).
Culture conditions
Further open issues are (i) seeding densities in a defined growth medium (Ben Azouna et al., 2012; Hagmann et al., 2013), (ii) specification of media supplements (Aldahmash et al., 2011; Bieback et al., 2012; Chimenti et al., 2014; Stern-Straeter et al., 2014), and (iii) atmospheric oxygen conditions during culture and handling (Grayson et al., 2006; Klepsch et al., 2013; Ito et al., 2015). Not solely in culture expansion, serum is also often used in cryopreservation. The use of animal-derived serum bears contamination risks and lot-to-lot variability. For clinical translation, it is therefore reasoned to replace animal-derived supplements (Tekkatte et al., 2011). Using autologous human serum has been proposed (Stute et al., 2004). Arguments such as high costs and the high likelihood of factors in the blood of donors, which may dominantly impact on MSC growth, greatly promoted the development of serum-free, chemically defined media (Mimura et al., 2011; Chase et al., 2012; Li et al., 2015). Alternatively, standardized human blood-derived products have been proposed (Díez et al., 2015; Riordan et al., 2015). Still unresolved and seemingly important in establishing MSC for therapeutic application is the definition of potency assays, that address patient's age.
Moving into clinics
In recent years, more than 500 clinical trials employing MSC for the treatment of various diseases have been registered worldwide (http://www.clinicaltrials.gov, 60 thereof in Europe www.clinicaltrialsregister.eu). Besides applications in musculoskeletal defects and trauma MSC are also widely tested in pathologies, which are of immunological etiology such as graft-vs.-host disease or multiple sclerosis, lupus, diabetes type I, and Crone's disease. The reason is, MSC exhibit dominant immune-modulatory properties (Castro-Manrreza and Montesinos, 2015). More and more details regarding the underlying molecular mechanisms and cellular interactions how MSC control immune competent cells are being unraveled (Glenn and Whartenby, 2014). MSCs are also tested for liver and heart pathologies as well as ocular diseases (Li et al., 2014b).
Clinical trial cohort variability
Many approaches are still exploratory and many ongoing clinical trials are still in Phase I Most strategies were carefully validated in diligently designed preclinical tests. However, commencing clinical trials firstly address safety issues thus rarely corroborating results cannot be expected. Early phase clinical studies also often comprise small heterogeneous groups exhibiting variant health status, age and ethnicity. Also sex-specific differences appear to be of relevance (Tajiri et al., 2014). For example, MSC from female bone marrow could be smaller and lower in number (Zanotti et al., 2014) but divided more rapidly than found for most male MSC. They had higher clonogenic activity and exhibited enhanced expression of the surface antigens, CD119 and CD130 (Siegel et al., 2013). Furthermore, functional differences were reported to be sexually dimorphic. Suppression of T-lymphocyte proliferation is more marked in females, while male-derived MSC possess a more robust osteogenic activity (Siegel et al., 2013; Ranganathan et al., 2014; Park et al., 2015). Female MSC showed a higher resistance in endotoxic and hypoxic injury models, inferring that improved survival in adverse micro-environments may be dependent on sex steroids. Indeed estrogen and estradiol exert a protective activity against apoptosis, favor proliferation, and delay the onset of senescence (Huang et al., 2013; Li et al., 2014c; Sung et al., 2015).
Most trials refrain from stratifying into age groups for obvious reasons of addressing a specific pathology rather than a gerontological principle. Animal trials are mostly done on young animals; clinical trials are rarely concerned with young or middle aged adults. MSC for animal experimentation are most often isolated from young animals, instead in humans autologous approaches are performed mostly in aged individuals. Further concerns are the greatly varying cell isolation and expansion procedures, as the outcome of a clinical trial is pertinently influenced by the quality of a cellular product. In fact, the population of cells harvested from bone marrow aspirates is very heterogeneous, consisting only of 0.001–0.01% mesenchymal precursor cells. To gain sufficient numbers of potential stem cells, the isolates are purified for subsequent in vitro expansion. At that point the uncertainty arises whether replicating cells accumulate damage which turns them senescent (Bonab et al., 2006). Suffice it to say that the recommendation is to restrict applications to replicating young MSC.
MSC aging: Cellular changes and determinants
First animal studies showed, already 10 years ago, that the transplantation of aged rat MSC was less effective (Zhang et al., 2005). Hence, the vexed question arose whether donor age influences the therapeutic efficacy in clinical trials (Wang et al., 2013b). MSC derived from old patients exhibit reduced proliferative capacity and in some cases show skewed multi-lineage differentiation potentials, telomere shortening, or DNA damage accumulation (Behrens et al., 2014; Efimenko et al., 2014; Kizilay Mancini et al., 2015; Reitinger et al., 2015). They also exhibit increased levels of reactive oxygen species and nitric oxide, lower superoxydismutase activity senescence-associated β-galactosidase activity, enlarged morphology, and p53 protein upregulation. These observations confirm the doubts that aged cells may only be acceptable for transplantation if specially treated or selected (Kornicka et al., 2015). In cases of autologous cell-based applications, constitution, and health status of the patient may pertinently impact on the therapeutic outcome. Changes in MSC morphology, proliferation capacity, senescence, and multi-lineage potential are linked to advanced age but could be also induced by several disease conditions, thus compromising their therapeutic potencies (Sethe et al., 2006; Choudhery et al., 2014; Escacena et al., 2015).
It is aged people, who are the primary targets for stem cell therapy. Provided putative deviations, procedures selecting for flawless cells are potentially required before applying aged cells in autologous therapy. Allogenic transplantation of stem cells derived from young donors are considered to potentially overcome aging-related limitations (Figure 1).
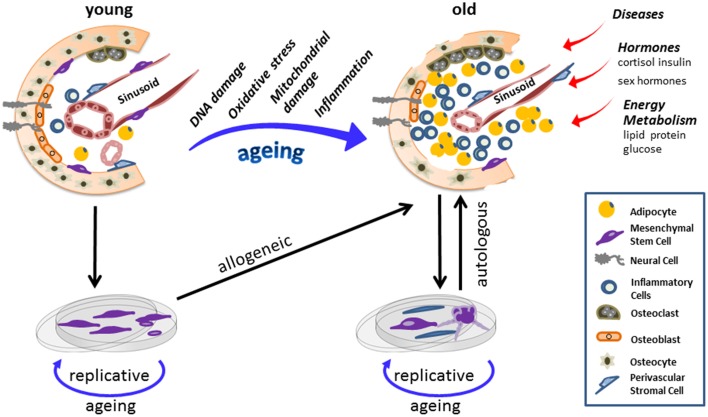
Stem-cell populations are maintained in “niches.” Stem cells and the corresponding niche entertain dynamic interactions controlling sustaining tissues regeneration and repair, yet also regulate somatic maintenance. Here a scheme of a bone marrow niche is provided and its potential changes as a consequence of the aging process. Chronological aging diminishes adult stem cells fitness and on the long run induces cellular and anatomical modifications within the niche. In old age mesenchymal stem cell (MSC) population has shrunken, while the number of inflammatory and fat cells increased to a large extent. Alongside, other extracellular factors also influence the aging process of stem cells together with their corresponding niche. Explanation of stem cells and in vitro amplifications adds risks of replicative aging to stem cell and progenitor fates. Hence when reintroducing cells in an autologous way, which is more likely undertaken as patients are more often of advanced age, chronologically old/replicative aged cells are applied. Conclusively, aberrations within a stem cell niche, as a consequence of aging, impose both chronological and replicative aging on therapeutic MSC, potentially compromising the success of autologous transplantation in aged donors.
It is further conceivable that the epigenetic status and/or aging as well as pathology-specific changes of cells may be different when isolating MSC from different tissues. It is currently unclear how to predict the optimal MSC source in order to warrant an optimal outcome (Golpanian et al., 2015). Interventions provoking rejuvenation of MSC have therefore been proposed. Extracellular factors, such as oxygen tension or redox status, exert effects on MSC aging (De Barros et al., 2013; Bigot et al., 2015; Sart et al., 2015).
Microenvironmental conditions, epigenetic processes including DNA methylation, telomerase activity, microRNA as well as specific growth factor signaling actively modulate MSC fate (Madonna et al., 2013; Jing et al., 2015; Oh et al., 2015; Okada et al., 2015). For their role in MSC biology, all these aspects provide challenging means to further enhance stem cell efficacy. Yet to date methods need to reliably prompt efficacy before being implemented in cell production at authorized manufacturing sites (Oh et al., 2014). Measuring the cellular fitness of MSC has been suggested being a invaluable prognostic value for enhancing the therapeutic success (Wagner et al., 2010). This complex question may however only be tackled by generating a comprehensive database for comparative purposes that stores information on source and MSC characteristics derived from different disease conditions. It is very likely that a pathological state perturbs the tissue milieu (niche) where MSC reside (Mastri et al., 2014). A systemically challenged micro-environment will impinge on the functional integrity of MSC, acknowledging the widely accepted principle of a stem cell niche (Krinner and Roeder, 2014). MSC niche interactions appear to be of mutual benefit since MSC release a wide range of bioactive factors that confer trophic and immune-modulatory effects, such as cytokines and distinct forms of miRNA and tRNA species (Hsiao et al., 2012; Baglio et al., 2015). More and more reports have unraveled the potent features of the MSC secretome, which now is thought to become by itself a regenerative therapeutical tool (Sdrimas and Kourembanas, 2014; Gallina et al., 2015; Succar et al., 2015; Tran and Damaser, 2015).
Considering the knowledge that the patient's disease state pertinently affects MSC functionality, the impact of medication on MSC, be it before or after implantation, is largely neglected and little data are available. MSC are greatly resistant to chemotherapy agents and therefore, therapies in patients undergoing cancer treatment are conceivable (Beane et al., 2014b; Bosco et al., 2015; Yoon et al., 2015) but have yet been not consistently successful (Buttiglieri et al., 2011; Choron et al., 2015). Medications, such as immune suppressants, glucocorticoids, psychopharmaceutics, and contrast agents may interfere with cell viability and proliferation capacity (Georgiou et al., 2012; Jansen Of Lorkeers et al., 2014; Schneider et al., 2015; Tang et al., 2015; Tsuji et al., 2015). These studies provided preliminary though valuable evidences, suggesting the need of more consistent drug interaction studies with special attention given to both short and long-term observations.
Conclusion
MSC are considered “work horses” for cell-based therapy. This is because very little ethical concerns have been raised and it is now widely accepted that MSC are largely resistant to malignant transformation. Being ubiquitously present in many tissues, MSC could be successfully isolated from cord blood, fat, skeletal muscles, dental pulp, and several other sources (Ogura et al., 2014). Despite all positive aspects and the remarkable progress in stem cell research, many decisive issues remain to be resolved. Difficult standardization on one hand, the persisting ambiguity regarding MSC-specific markers and the technical challenges with orthotopic transplantations are hampering experimental validations. It is important to unambiguously prove that stromal tissues of various origins contain stromal stem progenitor cells. In contrast to bone marrow-derived MSC, most experimental procedures aiming at identifying MSC in different tissues were solely based on in vitro data regarding non-clonal culture and multi-lineage differentiation. Therefore, the true stem cell character of these MSC has yet not be fully proven (Bhartiya, 2013). For these reasons, their physiology and functions in vivo are still scarcely known. Currently, information regarding biological and molecular features, as well as the dominant influences on their surrounding tissue environment, appears insufficient (Murray et al., 2014; New et al., 2015). In fact, sharing the same immune phenotype may be a deceptive indicator for predicting cellular function. Further “known unknowns” are details about migratory properties of MSC. MSC are likely incapable of entering the circulation (Hoogduijn et al., 2014). However, short-distance migration into adjacent tissues is conceivable (Vanden Berg-Foels, 2014), implying that a population of MSC in any given tissue might indeed represent a mixture of local and “migrant” MSC.
In light of these considerations, the question whether special MSC sources are putatively more potent in specific disease treatments requires in depth studies, addressing comparative measures of therapeutic efficacy and safety. Several studies albeit designed and performed in a comparable fashion reported that superficially equal MSC resulted in different outcomes in vivo (Meraviglia et al., 2014; Wang et al., 2014; Reinisch et al., 2015). To overcome the present discrepancies, US Food and Drug Administration (FDA) suggested building a database, in which data from MSC subjected to varying culture conditions, derived from diverse tissues, and donors should be compiled.
Once safety of MSC administration can be granted, the next step is to obtain consistent results on sustained curative benefit. Inconsistency or failure in clinical outcomes might not be solely related to MSC preparations, but also attributable to disparate therapeutic protocols. The optimal route of MSC delivery and dosage regime are still debated (Kean et al., 2013; Richardson et al., 2013; Chang et al., 2014; Yavagal et al., 2014). The success of a systemic delivery depends very much on the ability of MSC to home to the site of injury and to access target tissues in case of a damaged or still regenerating vascularization (Cerri et al., 2015). Efficacious outcomes after intravenous delivery have repeatedly been reported (Semedo et al., 2009; Cruz et al., 2015; Rapp et al., 2015). Needless to say that topic administration could be more successful (Antunes et al., 2014; Ishihara et al., 2014; Cerri et al., 2015; Huang et al., 2015).
Specification and definition of efficacy is another pending question to be answered. Multi-lineage differentiation in vitro has often been employed to deliberately grant in vivo efficacy. As the one has very little to do with the other, in particular because differentiation in vitro is performed under tightly controlled culture conditions, better methods have been conceived. In vivo, MSC differentiation or complex functions certainly depend on a plethora of parameters, which can be hardly reconstructed in vitro. Direct interactions with micro-vascular structures as well as with other cell types, such as endothelial cells appear to play stimulating and inducing roles (Chen et al., 2015; da Silva Meirelles et al., 2015; Tsai et al., 2015). Working along this line, the ability of MSC to suppress T- and B-lymphocytes proliferation and to produce lymphokines was exploited to predict MSC efficacy in vivo (Collins et al., 2014; Del Fattore et al., 2015). But once more controversial results have been reported (Sajic et al., 2012).
This pointed at establishing superior methods to verify the functional capacity of MSC prior clinical applications. Thus, tests in living animal were proposed to distinctly assay intrinsic capacities to differentiate into functional tissues or to exert other desired functions. Sequential heterotopic transplantation has proven to fulfill this need. Generation of heterotopic ossicles has demonstrated not only skeletogenic potentials of single clone progenitors but also self-renewal ability. It must be stressed that in vivo functional assays also provided means and measures to simultaneously acquire long-term safety data. There is a lack of suitable long-lived models, which would allow the sensitive risk assessment of MSC or its secretome in inducing malignancy (Bruno et al., 2014; Arango-Rodriguez et al., 2015; Schweizer et al., 2015).
A major caveat in most clinical MSC applications is the lack of long-term follow ups. Such studies would reveal those parameters that are essential in determining risk and safety issues in cell therapy. Despite some recent attempts (Brizuela et al., 2014; Caminal et al., 2014; Ciccocioppo et al., 2015; Daltro et al., 2015; Davatchi et al., 2015), we are currently far from a systematic and robust analysis. Only little attention has been given to acquire information on survival after implantation, on the impact of MSC cell therapy on other tissues or on unintended alterations in target tissue as well as in the tissue-borne MSC population itself. Implementation of MSC in therapies raises many practical questions which are beyond scientific consideration. Presently most trials are on a small-scale and mainly performed by academic centers. To acquire reliable and precise information on how to efficiently introduce MSC in clinical therapy, larger trials are needed with the active involvement of industry and the support of interdisciplinary teams.
Author contributions
All authors helped with drafting and revision of the article, GL completed the manuscript. All authors approved the submitted version.
Conflict of interest statement
The authors declare that the research was conducted in the absence of any commercial or financial relationships that could be construed as a potential conflict of interest.
Acknowledgments
We acknowledge financial support by the Open Access Publication Fund of University of Salzburg.
References
- Aldahmash A., Haack-Sørensen M., Al-Nbaheen M., Harkness L., Abdallah B. M., Kassem M. (2011). Human serum is as efficient as fetal bovine serum in supporting proliferation and differentiation of human multipotent stromal (mesenchymal) stem cells in vitro and in vivo. Stem Cell Rev. 7, 860–868. 10.1007/s12015-011-9274-2 [PubMed] [Cross Ref]
- Al-Nbaheen M., Vishnubalaji R., Ali D., Bouslimi A., Al-Jassir F., Megges M., et al. . (2013). Human stromal (mesenchymal) stem cells from bone marrow, adipose tissue and skin exhibit differences in molecular phenotype and differentiation potential. Stem Cell Rev. 9, 32–43. 10.1007/s12015-012-9365-8 [PMC free article] [PubMed] [Cross Ref]
- Antunes M. A., Abreu S. C., Cruz F. F., Teixeira A. C., Lopes-Pacheco M., Bandeira E., et al. . (2014). Effects of different mesenchymal stromal cell sources and delivery routes in experimental emphysema. Respir. Res. 15:118. 10.1186/s12931-014-0118-x [PMC free article] [PubMed] [Cross Ref]
- Arango-Rodriguez M. L., Ezquer F., Ezquer M., Conget P. (2015). Could cancer and infection be adverse effects of mesenchymal stromal cell therapy? World J. Stem Cells 7, 408–417. 10.4252/wjsc.v7.i2.408 [PMC free article] [PubMed] [Cross Ref]
- Baer P. C. (2014). Adipose -derived mesenchymal stromal/stem cells: an update on their phenotype in vivo and in vitro. World J. Stem Cells 6, 256–265. 10.4252/wjsc.v6.i3.256 [PMC free article] [PubMed] [Cross Ref]
- Baglio S. R., Rooijers K., Koppers-Lalic D., Verweij F. J., Pérez Lanzón M., Zini N., et al. . (2015). Human bone marrow- and adipose-mesenchymal stem cells secrete exosomes enriched in distinctive miRNA and tRNA species. Stem Cell Res. Ther. 6, 127. 10.1186/s13287-015-0116-z [PMC free article] [PubMed] [Cross Ref]
- Bajek A., Czerwinski M., Olkowska J., Gurtowska N., Kloskowski T., Drewa T. (2012). Does aging of mesenchymal stem cells limit their potential application in clinical practice? Aging Clin. Exp. Res. 24, 404–411. 10.3275/8424 [PubMed] [Cross Ref]
- Batsali A. K., Kastrinaki M. C., Papadaki H. A., Pontikoglou C. (2013). Mesenchymal stem cells derived from Wharton's Jelly of the umbilical cord: biological properties and emerging clinical applications. Curr. Stem Cell Res. Ther. 8, 144–155. 10.2174/1574888X11308020005 [PubMed] [Cross Ref]
- Beane O. S., Fonseca V. C., Cooper L. L., Koren G., Darling E. M. (2014a). Impact of aging on the regenerative properties of bone marrow-, muscle-, and adipose-derived mesenchymal stem/stromal cells. PLoS ONE 9:e115963. 10.1371/journal.pone.0115963 [PMC free article] [PubMed] [Cross Ref]
- Beane O. S., Fonseca V. C., Darling E. M. (2014b). Adipose -derived stem cells retain their regenerative potential after methotrexate treatment. Exp. Cell Res. 327, 222–233. 10.1016/j.yexcr.2014.06.015 [PMC free article] [PubMed] [Cross Ref]
- Behrens A., van Deursen J. M., Rudolph K. L., Schumacher B. (2014). Impact of genomic damage and ageing on stem cell function. Nat. Cell Biol. 16, 201–207. 10.1038/ncb2928 [PMC free article] [PubMed] [Cross Ref]
- Ben Azouna N., Jenhani F., Regaya Z., Berraeis L., Ben Othman T., Ducrocq E., et al. . (2012). Phenotypical and functional characteristics of mesenchymal stem cells from bone marrow: comparison of culture using different media supplemented with human platelet lysate or fetal bovine serum. Stem Cell Res. Ther. 3, 6. 10.1186/scrt97 [PMC free article] [PubMed] [Cross Ref]
- Bethel M., Chitteti B. R., Srour E. F., Kacena M. A. (2013). The changing balance between osteoblastogenesis and adipogenesis in aging and its impact on hematopoiesis. Curr. Osteoporos. Rep. 11, 99–106. 10.1007/s11914-013-0135-6 [PMC free article] [PubMed] [Cross Ref]
- Bhartiya D. (2013). Are mesenchymal cells indeed pluripotent stem cells or just stromal cells? OCT-4 and VSELs biology has led to better understanding. Stem Cells Int. 2013:547501. 10.1155/2013/547501 [PMC free article] [PubMed] [Cross Ref]
- Bianco P. (2014). “Mesenchymal” stem cells. Annu. Rev. Cell Dev. Biol. 30, 677–704. 10.1146/annurev-cellbio-100913-013132 [PubMed] [Cross Ref]
- Bieback K., Hecker A., Schlechter T., Hofmann I., Brousos N., Redmer T., et al. . (2012). Replicative aging and differentiation potential of human adipose tissue-derived mesenchymal stromal cells expanded in pooled human or fetal bovine serum. Cytotherapy 14, 570–583. 10.3109/14653249.2011.652809 [PubMed] [Cross Ref]
- Bigot N., Mouche A., Preti M., Loisel S., Renoud M. L., Le Guével R., et al. . (2015). Hypoxia differentially modulates the genomic stability of clinical-grade ADSCs and BM-MSCs in long-term culture. Stem Cells. . [Epub ahead of print]. 10.1002/stem.2195 [PubMed] [Cross Ref]
- Bonab M. M., Alimoghaddam K., Talebian F., Ghaffari S. H., Ghavamzadeh A., Nikbin B. (2006). Aging of mesenchymal stem cell in vitro. BMC Cell Biol. 7:14. 10.1186/1471-2121-7-14 [PMC free article] [PubMed] [Cross Ref]
- Bosco D. B., Kenworthy R., Zorio D. A., Sang Q. X. (2015). Human mesenchymal stem cells are resistant to Paclitaxel by adopting a non-proliferative fibroblastic state. PLoS ONE 10:e0128511. 10.1371/journal.pone.0128511 [PMC free article] [PubMed] [Cross Ref]
- Brizuela A., Martín N., Fernández-Gonzalez F. J., Larrazábal C., Anta A. (2014). Osteotome sinus floor elevation without grafting material: results of a 2-year prospective study. J. Clin. Exp. Dent. 6, e479–e484. 10.4317/jced.51576 [PMC free article] [PubMed] [Cross Ref]
- Bruno S., Collino F., Iavello A., Camussi G. (2014). Effects of mesenchymal stromal cell-derived extracellular vesicles on tumor growth. Front. Immunol. 5:382. 10.3389/fimmu.2014.00382 [PMC free article] [PubMed] [Cross Ref]
- Busser H., Najar M., Raicevic G., Pieters K., Velez Pombo R., Philippart P., et al. . (2015). Isolation and characterization of human mesenchymal stromal cell subpopulations: comparison of bone marrow and adipose tissue. Stem Cells Dev. 24, 2142–2157. 10.1089/scd.2015.0172 [PubMed] [Cross Ref]
- Buttiglieri S., Ruella M., Risso A., Spatola T., Silengo L., Avvedimento E. V., et al. . (2011). The aging effect of chemotherapy on cultured human mesenchymal stem cells. Exp. Hematol. 39, 1171–1181. 10.1016/j.exphem.2011.08.009 [PubMed] [Cross Ref]
- Caminal M., Fonseca C., Peris D., Moll X., Rabanal R. M., Barrachina J., et al. . (2014). Use of a chronic model of articular cartilage and meniscal injury for the assessment of long-term effects after autologous mesenchymal stromal cell treatment in sheep. N. Biotechnol. 31, 492–498. 10.1016/j.nbt.2014.07.004 [PubMed] [Cross Ref]
- Castro-Malaspina H., Gay R. E., Resnick G., Kapoor N., Meyers P., Chiarieri D., et al. . (1980). Characterization of human bone marrow fibroblast colony-forming cells (CFU-F) and their progeny. Blood 56, 289–301. [PubMed]
- Castro-Manrreza M. E., Montesinos J. J. (2015). Immunoregulation by mesenchymal stem cells: biological aspects and clinical applications. J. Immunol. Res. 2015:394917. 10.1155/2015/394917 [PMC free article] [PubMed] [Cross Ref]
- Cerri S., Greco R., Levandis G., Ghezzi C., Mangione A. S., Fuzzati-Armentero M. T., et al. . (2015). Intracarotid infusion of mesenchymal stem cells in an animal model of parkinson's disease, focusing on cell distribution and neuroprotective and behavioral effects. Stem Cells Transl. Med. 4, 1073–1085. 10.5966/sctm.2015-0023 [PMC free article] [PubMed] [Cross Ref]
- Chang Y. S., Ahn S. Y., Yoo H. S., Sung S. I., Choi S. J., Oh W. I., et al. . (2014). Mesenchymal stem cells for bronchopulmonary dysplasia: phase 1 dose-escalation clinical trial. J. Pediatr. 164, 966–972.e6. 10.1016/j.jpeds.2013.12.011 [PubMed] [Cross Ref]
- Chase L. G., Yang S., Zachar V., Yang Z., Lakshmipathy U., Bradford J., et al. . (2012). Development and characterization of a clinically compliant xeno-free culture medium in good manufacturing practice for human multipotent mesenchymal stem cells. Stem Cells Transl. Med. 1, 750–758. 10.5966/sctm.2012-0072 [PMC free article] [PubMed] [Cross Ref]
- Chen C. W., Corselli M., Péault B., Huard J. (2012). Human blood-vessel-derived stem cells for tissue repair and regeneration. J. Biomed. Biotechnol. 2012:597439. 10.1155/2012/597439 [PMC free article] [PubMed] [Cross Ref]
- Chen W. C., Péault B., Huard J. (2015). Regenerative translation of human blood-vessel-derived MSC precursors. Stem Cells Int. 2015:375187. 10.1155/2015/375187 [PMC free article] [PubMed] [Cross Ref]
- Chimenti I., Gaetani R., Forte E., Angelini F., De Falco E., Zoccai G. B., et al. . (2014). Serum and supplement optimization for EU GMP-compliance in cardiospheres cell culture. J. Cell. Mol. Med. 18, 624–634. 10.1111/jcmm.12210 [PMC free article] [PubMed] [Cross Ref]
- Choron R. L., Chang S., Khan S., Villalobos M. A., Zhang P., Carpenter J. P., et al. . (2015). Paclitaxel impairs adipose stem cell proliferation and differentiation. J. Surg. Res. 196, 404–415. 10.1016/j.jss.2015.03.026 [PMC free article] [PubMed] [Cross Ref]
- Choudhery M. S., Badowski M., Muise A., Pierce J., Harris D. T. (2014). Donor age negatively impacts adipose tissue-derived mesenchymal stem cell expansion and differentiation. J. Transl. Med. 12:8. 10.1186/1479-5876-12-8 [PMC free article] [PubMed] [Cross Ref]
- Ciccocioppo R., Gallia A., Sgarella A., Kruzliak P., Gobbi P. G., Corazza G. R. (2015). Long -term follow-up of crohn disease fistulas after local injections of bone marrow-derived mesenchymal stem cells. Mayo Clin. Proc. 90, 747–755. 10.1016/j.mayocp.2015.03.023 [PubMed] [Cross Ref]
- Collins E., Gu F., Qi M., Molano I., Ruiz P., Sun L., et al. . (2014). Differential efficacy of human mesenchymal stem cells based on source of origin. J. Immunol. 193, 4381–4390. 10.4049/jimmunol.1401636 [PMC free article] [PubMed] [Cross Ref]
- Cruz F. F., Borg Z. D., Goodwin M., Sokocevic D., Wagner D., McKenna D. H., et al. . (2015). Freshly thawed and continuously cultured human bone marrow-derived mesenchymal stromal cells comparably ameliorate allergic airways inflammation in immunocompetent mice. Stem Cells Transl. Med. 4, 615–624. 10.5966/sctm.2014-0268 [PMC free article] [PubMed] [Cross Ref]
- Cuthbert R. J., Giannoudis P. V., Wang X. N., Nicholson L., Pawson D., Lubenko A., et al. . (2015). Examining the feasibility of clinical grade CD271+ enrichment of mesenchymal stromal cells for bone regeneration. PLoS ONE 10:e0117855. 10.1371/journal.pone.0117855 [PMC free article] [PubMed] [Cross Ref]
- da Silva Meirelles L., Malta T. M., de Deus Wagatsuma V. M., Palma P. V., Araújo A. G., Ribeiro Malmegrim K. C., et al. . (2015). Cultured human adipose tissue pericytes and mesenchymal stromal cells display a very similar gene expression profile. Stem Cells Dev. 24, 2822–2840. 10.1089/scd.2015.0153 [PMC free article] [PubMed] [Cross Ref]
- Daltro G. C., Fortuna V., de Souza E. S., Salles M. M., Carreira A. C., Meyer R., et al. . (2015). Efficacy of autologous stem cell-based therapy for osteonecrosis of the femoral head in sickle cell disease: a five-year follow-up study. Stem Cell Res. Ther. 6, 110. 10.1186/s13287-015-0105-2 [PMC free article] [PubMed] [Cross Ref]
- Davatchi F., Sadeghi Abdollahi B., Mohyeddin M., Nikbin B. (2015). Mesenchymal stem cell therapy for knee osteoarthritis: 5 years follow-up of three patients. Int. J. Rheum. Dis. . [Epub ahead of print]. 10.1111/1756-185X.12670 [PubMed] [Cross Ref]
- De Barros S., Dehez S., Arnaud E., Barreau C., Cazavet A., Perez G., et al. . (2013). Aging -related decrease of human ASC angiogenic potential is reversed by hypoxia preconditioning through ROS production. Mol. Ther. 21, 399–408. 10.1038/mt.2012.213 [PMC free article] [PubMed] [Cross Ref]
- Del Fattore A., Luciano R., Pascucci L., Goffredo B. M., Giorda E., Scapaticci M., et al. . (2015). Immunoregulatory effects of Mesenchymal stem cell-derived extracellular vesicles on T lymphocytes. Cell Transplant. . [Epub ahead of print]. 10.3727/096368915X687543 [PubMed] [Cross Ref]
- Deschaseaux F., Gindraux F., Saadi R., Obert L., Chalmers D., Herve P. (2003). Direct selection of human bone marrow mesenchymal stem cells using an anti-CD49a antibody reveals their CD45med, low phenotype. Br. J. Haematol. 122, 506–517. 10.1046/j.1365-2141.2003.04469.x [PubMed] [Cross Ref]
- Díez J. M., Bauman E., Gajardo R., Jorquera J. I. (2015). Culture of human mesenchymal stem cells using a candidate pharmaceutical grade xeno-free cell culture supplement derived from industrial human plasma pools. Stem Cell Res. Ther. 6, 28. 10.1186/s13287-015-0016-2 [PMC free article] [PubMed] [Cross Ref]
- Dominici M., Le Blanc K., Mueller I., Slaper-Cortenbach I., Marini F., Krause D., et al. . (2006). Minimal criteria for defining multipotent mesenchymal stromal cells. The international society for cellular therapy position statement. Cytotherapy 8, 315–317. 10.1080/14653240600855905 [PubMed] [Cross Ref]
- Efimenko A., Dzhoyashvili N., Kalinina N., Kochegura T., Akchurin R., Tkachuk V., et al. . (2014). Adipose -derived mesenchymal stromal cells from aged patients with coronary artery disease keep mesenchymal stromal cell properties but exhibit characteristics of aging and have impaired angiogenic potential. Stem Cells Transl. Med. 3, 32–41. 10.5966/sctm.2013-0014 [PMC free article] [PubMed] [Cross Ref]
- Escacena N., Quesada-Hernández E., Capilla-Gonzalez V., Soria B., Hmadcha A. (2015). Bottlenecks in the efficient use of advanced therapy medicinal products based on mesenchymal stromal cells. Stem Cells Int. 2015:895714. 10.1155/2015/895714 [PMC free article] [PubMed] [Cross Ref]
- Feng J., Mantesso A., De Bari C., Nishiyama A., Sharpe P. T. (2011). Dual origin of mesenchymal stem cells contributing to organ growth and repair. Proc. Natl. Acad. Sci. U.S.A. 108, 6503–6508. 10.1073/pnas.1015449108 [PMC free article] [PubMed] [Cross Ref]
- Friedenstein A. J. (1976). Precursor cells of mechanocytes. Int. Rev. Cytol. 47, 327–359. 10.1016/S0074-7696(08)60092-3 [PubMed] [Cross Ref]
- Gallina C., Turinetto V., Giachino C. (2015). A new paradigm in cardiac regeneration: the mesenchymal stem cell secretome. Stem Cells Int. 2015:765846. 10.1155/2015/765846 [PMC free article] [PubMed] [Cross Ref]
- Georgiou K. R., Hui S. K., Xian C. J. (2012). Regulatory pathways associated with bone loss and bone marrow adiposity caused by aging, chemotherapy, glucocorticoid therapy and radiotherapy. Am. J. Stem Cells 1, 205–224. [PMC free article] [PubMed]
- Glenn J. D., Whartenby K. A. (2014). Mesenchymal stem cells: emerging mechanisms of immunomodulation and therapy. World J. Stem Cells 6, 526–539. 10.4252/wjsc.v6.i5.526 [PMC free article] [PubMed] [Cross Ref]
- Golpanian S., El-Khorazaty J., Mendizabal A., DiFede D. L., Suncion V. Y., Karantalis V., et al. . (2015). Effect of aging on human mesenchymal stem cell therapy in ischemic cardiomyopathy patients. J. Am. Coll. Cardiol. 65, 125–132. 10.1016/j.jacc.2014.10.040 [PMC free article] [PubMed] [Cross Ref]
- Grayson W. L., Zhao F., Izadpanah R., Bunnell B., Ma T. (2006). Effects of hypoxia on human mesenchymal stem cell expansion and plasticity in 3D constructs. J. Cell. Physiol. 207, 331–339. 10.1002/jcp.20571 [PubMed] [Cross Ref]
- Gronthos S., Zannettino A. C., Graves S. E., Ohta S., Hay S. J., Simmons P. J. (1999). Differential cell surface expression of the STRO-1 and alkaline phosphatase antigens on discrete developmental stages in primary cultures of human bone cells. J. Bone Miner. Res. 14, 47–56. 10.1359/jbmr.1999.14.1.47 [PubMed] [Cross Ref]
- Hagmann S., Moradi B., Frank S., Dreher T., Kämmerer P. W., Richter W., et al. . (2013). Different culture media affect growth characteristics, surface marker distribution and chondrogenic differentiation of human bone marrow-derived mesenchymal stromal cells. BMC Musculoskelet. Disord. 14:223. 10.1186/1471-2474-14-223 [PMC free article] [PubMed] [Cross Ref]
- Hanley P. J., Mei Z., Durett A. G., Cabreira-Hansen Mda G., Klis M., Li W., et al. . (2014). Efficient manufacturing of therapeutic mesenchymal stromal cells with the use of the Quantum Cell Expansion System. Cytotherapy 16, 1048–1058. 10.1016/j.jcyt.2014.01.417 [PMC free article] [PubMed] [Cross Ref]
- Hao L., Sun H., Wang J., Wang T., Wang M., Zou Z. (2012). Mesenchymal stromal cells for cell therapy: besides supporting hematopoiesis. Int. J. Hematol. 95, 34–46. 10.1007/s12185-011-0991-8 [PubMed] [Cross Ref]
- Hoogduijn M. J., Verstegen M. M., Engela A. U., Korevaar S. S., Roemeling-van Rhijn M., Merino A., et al. . (2014). No evidence for circulating mesenchymal stem cells in patients with organ injury. Stem Cells Dev. 23, 2328–2335. 10.1089/scd.2014.0269 [PubMed] [Cross Ref]
- Hsiao S. T., Asgari A., Lokmic Z., Sinclair R., Dusting G. J., Lim S. Y., et al. . (2012). Comparative analysis of paracrine factor expression in human adult mesenchymal stem cells derived from bone marrow, adipose, and dermal tissue. Stem Cells Dev. 21, 2189–2203. 10.1089/scd.2011.0674 [PMC free article] [PubMed] [Cross Ref]
- Huang C. K., Tsai M. Y., Luo J., Kang H. Y., Lee S. O., Chang C. (2013). Suppression of androgen receptor enhances the self-renewal of mesenchymal stem cells through elevated expression of EGFR. Biochim. Biophys. Acta 1833, 1222–1234. 10.1016/j.bbamcr.2013.01.007 [PMC free article] [PubMed] [Cross Ref]
- Huang S., Xu L., Zhang Y., Sun Y., Li G. (2015). Systemic and local administration of allogeneic bone marrow derived mesenchymal stem cells promotes fracture healing in rats. Cell Transplant. . [Epub ahead of print]. 10.3727/096368915X687219 [PubMed] [Cross Ref]
- Ishihara A., Ohmine K., Weisbrode S. E., Bertone A. L. (2014). Effect of intra-medullar and intra-venous infusions of mesenchymal stem cells on cell engraftment by in-vivo cell tracking and osteoinductivity in rabbit long bones: a pilot study. Orthop. Muscular. Syst. 3:1000172. 10.4172/2161-0533.1000172 [PMC free article] [PubMed] [Cross Ref]
- Ito K., Aoyama T., Fukiage K., Otsuka S., Furu M., Jin Y., et al. . (2010). A novel method to isolate mesenchymal stem cells from bone marrow in a closed system using a device made by nonwoven fabric. Tissue Eng. Part C Methods 16, 81–91. 10.1089/ten.tec.2008.0693 [PubMed] [Cross Ref]
- Ito A., Aoyama T., Yoshizawa M., Nagai M., Tajino J., Yamaguchi S., et al. . (2015). The effects of short-term hypoxia on human mesenchymal stem cell proliferation, viability and p16(INK4A) mRNA expression: investigation using a simple hypoxic culture system with a deoxidizing agent. J. Stem Cells Regen. Med. 11, 25–31. [PMC free article] [PubMed]
- Jansen Of Lorkeers S. J., Hart E., Tang X. L., Chamuleau M. E., Doevendans P. A., Bolli R., et al. . (2014). Cyclosporin in cell therapy for cardiac regeneration. J. Cardiovasc. Transl. Res. 7, 475–482. 10.1007/s12265-014-9570-8 [PMC free article] [PubMed] [Cross Ref]
- Jing W., Smith A. A., Liu B., Li J., Hunter D. J., Dhamdhere G., et al. . (2015). Reengineering autologous bone grafts with the stem cell activator WNT3A. Biomaterials 47, 29–40. 10.1016/j.biomaterials.2014.12.014 [PubMed] [Cross Ref]
- Kaiser S., Hackanson B., Follo M., Mehlhorn A., Geiger K., Ihorst G., et al. . (2007). BM cells giving rise to MSC in culture have a heterogeneous CD34 and CD45 phenotype. Cytotherapy 9, 439–450. 10.1080/14653240701358445 [PubMed] [Cross Ref]
- Kean T. J., Lin P., Caplan A. I., Dennis J. E. (2013). MSCs: delivery routes and engraftment, cell-targeting strategies, and immune modulation. Stem Cells Int. 2013:732742. 10.1155/2013/732742 [PMC free article] [PubMed] [Cross Ref]
- Kerk D. K., Henry E. A., Eaves A. C., Eaves C. J. (1985). Two classes of primitive pluripotent hemopoietic progenitor cells: separation by adherence. J. Cell. Physiol. 125, 127–134. 10.1002/jcp.1041250117 [PubMed] [Cross Ref]
- Kizilay Mancini O., Shum-Tim D., Stochaj U., Correa J. A., Colmegna I. (2015). Age, atherosclerosis and type 2 diabetes reduce human mesenchymal stromal cell-mediated T-cell suppression. Stem Cell Res. Ther. 6, 140. 10.1186/s13287-015-0127-9 [PMC free article] [PubMed] [Cross Ref]
- Klepsch S., Jamnig A., Trimmel D., Schimke M., Kapferer W., Brunauer R., et al. . (2013). Isolation of mesenchymal stem cells from human bone and long-term cultivation under physiologic oxygen conditions. Methods Mol. Biol. 976, 99–109. 10.1007/978-1-62703-317-6_8 [PubMed] [Cross Ref]
- Kornicka K., Marycz K., Tomaszewski K. A., Maredziak M., Smieszek A. (2015). The effect of age on osteogenic and adipogenic differentiation potential of human adipose derived stromal stem cells (hASCs) and the impact of stress factors in the course of the differentiation process. Oxid. Med. Cell. Longev. 2015:309169. 10.1155/2015/309169 [PMC free article] [PubMed] [Cross Ref]
- Krinner A., Roeder I. (2014). Quantification and modeling of stem cell-niche interaction. Adv. Exp. Med. Biol. 844, 11–36. 10.1007/978-1-4939-2095-2_2 [PubMed] [Cross Ref]
- Kuznetsov S. A., Krebsbach P. H., Satomura K., Kerr J., Riminucci M., Benayahu D., et al. . (1997). Single-colony derived strains of human marrow stromal fibroblasts form bone after transplantation in vivo. J. Bone Miner. Res. 12, 1335–1347. 10.1359/jbmr.1997.12.9.1335 [PubMed] [Cross Ref]
- Landgraf K., Brunauer R., Lepperdinger G., Grubeck-Loebenstein B. (2011). The suppressive effect of mesenchymal stromal cells on T cell proliferation is conserved in old age. Transpl. Immunol. 25, 167–172. 10.1016/j.trim.2011.06.007 [PubMed] [Cross Ref]
- Law S., Chaudhuri S. (2013). Mesenchymal stem cell and regenerative medicine: regeneration versus immunomodulatory challenges. Am. J. Stem Cells 2, 22–38. [PMC free article] [PubMed]
- Li C. Y., Wu X. Y., Tong J. B., Yang X. X., Zhao J. L., Zheng Q. F., et al. . (2015). Comparative analysis of human mesenchymal stem cells from bone marrow and adipose tissue under xeno-free conditions for cell therapy. Stem Cell Res. Ther. 6, 55. 10.1186/s13287-015-0066-5 [PMC free article] [PubMed] [Cross Ref]
- Li L., Guo Y., Zhai H., Yin Y., Zhang J., Chen H., et al. . (2014a). Aging increases the susceptivity of MSCs to reactive oxygen species and impairs their therapeutic potency for myocardial infarction. PLoS ONE 9:e111850. 10.1371/journal.pone.0111850 [PMC free article] [PubMed] [Cross Ref]
- Li M. D., Atkins H., Bubela T. (2014b). The global landscape of stem cell clinical trials. Regen. Med. 9, 27–39. 10.2217/rme.13.80 [PubMed] [Cross Ref]
- Li Q., Zhang X., Peng Y., Chai H., Xu Y., Wei J., et al. . (2013). Comparison of the sorting efficiency and influence on cell function between the sterile flow cytometry and immunomagnetic bead purification methods. Prep. Biochem. Biotechnol. 43, 197–206. 10.1080/10826068.2012.719846 [PubMed] [Cross Ref]
- Li Y., Yan M., Wang Z., Zheng Y., Li J., Ma S., et al. . (2014c). 17beta-estradiol promotes the odonto/osteogenic differentiation of stem cells from apical papilla via mitogen-activated protein kinase pathway. Stem Cell Res Ther 5, 125. 10.1186/scrt515 [PMC free article] [PubMed] [Cross Ref]
- Lin G., Garcia M., Ning H., Banie L., Guo Y. L., Lue T. F., et al. . (2008). Defining stem and progenitor cells within adipose tissue. Stem Cells Dev. 17, 1053–1063. 10.1089/scd.2008.0117 [PMC free article] [PubMed] [Cross Ref]
- Lin G., Liu G., Banie L., Wang G., Ning H., Lue T. F., et al. . (2011). Tissue distribution of mesenchymal stem cell marker Stro-1. Stem Cells Dev. 20, 1747–1752. 10.1089/scd.2010.0564 [PMC free article] [PubMed] [Cross Ref]
- Madonna R., Taylor D. A., Geng Y. J., De Caterina R., Shelat H., Perin E. C., et al. . (2013). Transplantation of mesenchymal cells rejuvenated by the overexpression of telomerase and myocardin promotes revascularization and tissue repair in a murine model of hindlimb ischemia. Circ. Res. 113, 902–914. 10.1161/CIRCRESAHA.113.301690 [PubMed] [Cross Ref]
- Mark P., Kleinsorge M., Gaebel R., Lux C. A., Toelk A., Pittermann E., et al. . (2013). Human mesenchymal stem cells display reduced expression of CD105 after culture in serum-free medium. Stem Cells Int. 2013:698076. 10.1155/2013/698076 [PMC free article] [PubMed] [Cross Ref]
- Mastri M., Lin H., Lee T. (2014). Enhancing the efficacy of mesenchymal stem cell therapy. World J. Stem Cells 6, 82–93. 10.4252/wjsc.v6.i2.82 [PMC free article] [PubMed] [Cross Ref]
- Meraviglia V., Azzimato V., Piacentini L., Chiesa M., Kesharwani R. K., Frati C., et al. . (2014). Syngeneic cardiac and bone marrow stromal cells display tissue-specific microRNA signatures and microRNA subsets restricted to diverse differentiation processes. PLoS ONE 9:e107269. 10.1371/journal.pone.0107269 [PMC free article] [PubMed] [Cross Ref]
- Mimura S., Kimura N., Hirata M., Tateyama D., Hayashida M., Umezawa A., et al. . (2011). Growth factor-defined culture medium for human mesenchymal stem cells. Int. J. Dev. Biol. 55, 181–187. 10.1387/ijdb.103232sm [PubMed] [Cross Ref]
- Murray I. R., West C. C., Hardy W. R., James A. W., Park T. S., Nguyen A., et al. . (2014). Natural history of mesenchymal stem cells, from vessel walls to culture vessels. Cell. Mol. Life Sci. 71, 1353–1374. 10.1007/s00018-013-1462-6 [PubMed] [Cross Ref]
- New S. E., Alvarez-Gonzalez C., Vagaska B., Gomez S. G., Bulstrode N. W., Madrigal A., et al. . (2015). A matter of identity - Phenotype and differentiation potential of human somatic stem cells. Stem Cell Res. 15, 1–13. 10.1016/j.scr.2015.04.003 [PubMed] [Cross Ref]
- Ning H., Lin G., Lue T. F., Lin C. S. (2011). Mesenchymal stem cell marker Stro-1 is a 75 kd endothelial antigen. Biochem. Biophys. Res. Commun. 413, 353–357. 10.1016/j.bbrc.2011.08.104 [PMC free article] [PubMed] [Cross Ref]
- Nold P., Brendel C., Neubauer A., Bein G., Hackstein H. (2013). Good manufacturing practice-compliant animal-free expansion of human bone marrow derived mesenchymal stroma cells in a closed hollow-fiber-based bioreactor. Biochem. Biophys. Res. Commun. 430, 325–330. 10.1016/j.bbrc.2012.11.001 [PubMed] [Cross Ref]
- Ogura F., Wakao S., Kuroda Y., Tsuchiyama K., Bagheri M., Heneidi S., et al. . (2014). Human adipose tissue possesses a unique population of pluripotent stem cells with nontumorigenic and low telomerase activities: potential implications in regenerative medicine. Stem Cells Dev. 23, 717–728. 10.1089/scd.2013.0473 [PubMed] [Cross Ref]
- Oh J., Lee Y. D., Wagers A. J. (2014). Stem cell aging: mechanisms, regulators and therapeutic opportunities. Nat. Med. 20, 870–880. 10.1038/nm.3651 [PMC free article] [PubMed] [Cross Ref]
- Oh Y. S., Jeong S. G., Cho G. W. (2015). Anti-senescence effects of DNA methyltransferase inhibitor RG108 in human bone marrow mesenchymal stromal cells. Biotechnol. Appl. Biochem. 62, 583–590. 10.1002/bab.1393 [PubMed] [Cross Ref]
- Okada M., Kim H. W., Matsu-Ura K., Wang Y. G., Xu M., Ashraf M. (2015). Abrogation of age-induced microRNA-195 rejuvenates the senescent mesenchymal stem cells by reactivating telomerase. Stem Cells. . [Epub ahead of print]. 10.1002/stem.2211 [PubMed] [Cross Ref]
- Otsuru S., Hofmann T. J., Olson T. S., Dominici M., Horwitz E. M. (2013). Improved isolation and expansion of bone marrow mesenchymal stromal cells using a novel marrow filter device. Cytotherapy 15, 146–153. 10.1016/j.jcyt.2012.10.012 [PubMed] [Cross Ref]
- Otsuru S., Hofmann T. J., Raman P., Olson T. S., Guess A. J., Dominici M., et al. . (2015). Genomic and functional comparison of mesenchymal stromal cells prepared using two isolation methods. Cytotherapy 17, 262–270. 10.1016/j.jcyt.2014.10.013 [PubMed] [Cross Ref]
- Owen M., Friedenstein A. J. (1988). Stromal stem cells: marrow-derived osteogenic precursors. Ciba Found. Symp. 136, 42–60. [PubMed]
- Park S. S., Beyer R. P., Smyth M. D., Clarke C. M., Timms A. E., Bammler T. K., et al. . (2015). Osteoblast differentiation profiles define sex specific gene expression patterns in craniosynostosis. Bone 76, 169–176. 10.1016/j.bone.2015.03.001 [PMC free article] [PubMed] [Cross Ref]
- Patrikoski M., Sivula J., Huhtala H., Helminen M., Salo F., Mannerström B., et al. . (2014). Different culture conditions modulate the immunological properties of adipose stem cells. Stem Cells Transl. Med. 3, 1220–1230. 10.5966/sctm.2013-0201 [PMC free article] [PubMed] [Cross Ref]
- Pontikoglou C., Deschaseaux F., Sensebé L., Papadaki H. A. (2011). Bone marrow mesenchymal stem cells: biological properties and their role in hematopoiesis and hematopoietic stem cell transplantation. Stem Cell Rev. 7, 569–589. 10.1007/s12015-011-9228-8 [PubMed] [Cross Ref]
- Rada T., Gomes M. E., Reis R. L. (2011). A novel method for the isolation of subpopulations of rat adipose stem cells with different proliferation and osteogenic differentiation potentials. J. Tissue Eng. Regen. Med. 5, 655–664. 10.1002/term.364 [PubMed] [Cross Ref]
- Ranganathan K., Peterson J., Eboda O., Agarwal S., Buchman S., Cederna P., et al. . (2014). Abstract 133: gender differences in heterotopic ossification. Plast. Reconstr. Surg. 133, 149. 10.1097/01.prs.0000444960.44044.44 [PubMed] [Cross Ref]
- Rapp A. E., Bindl R., Heilmann A., Erbacher A., Müller I., Brenner R. E., et al. . (2015). Systemic mesenchymal stem cell administration enhances bone formation in fracture repair but not load-induced bone formation. Eur. Cell. Mater. 29, 22–34. [PubMed]
- Rasini V., Dominici M., Kluba T., Siegel G., Lusenti G., Northoff H., et al. . (2013). Mesenchymal stromal/stem cells markers in the human bone marrow. Cytotherapy 15, 292–306. 10.1016/j.jcyt.2012.11.009 [PubMed] [Cross Ref]
- Reinisch A., Etchart N., Thomas D., Hofmann N. A., Fruehwirth M., Sinha S., et al. . (2015). Epigenetic and in vivo comparison of diverse MSC sources reveals an endochondral signature for human hematopoietic niche formation. Blood 125, 249–260. 10.1182/blood-2014-04-572255 [PMC free article] [PubMed] [Cross Ref]
- Reitinger S., Schimke M., Klepsch S., de Sneeuw S., Yani S. L., Gaßner R., et al. . (2015). Systemic impact molds mesenchymal stromal/stem cell aging. Transfus. Apher. Sci. 52, 285–289. 10.1016/j.transci.2015.04.008 [PubMed] [Cross Ref]
- Richardson J. D., Bertaso A. G., Psaltis P. J., Frost L., Carbone A., Paton S., et al. . (2013). Impact of timing and dose of mesenchymal stromal cell therapy in a preclinical model of acute myocardial infarction. J. Card. Fail. 19, 342–353. 10.1016/j.cardfail.2013.03.011 [PubMed] [Cross Ref]
- Riekstina U., Muceniece R., Cakstina I., Muiznieks I., Ancans J. (2008). Characterization of human skin-derived mesenchymal stem cell proliferation rate in different growth conditions. Cytotechnology 58, 153–162. 10.1007/s10616-009-9183-2 [PMC free article] [PubMed] [Cross Ref]
- Riordan N. H., Madrigal M., Reneau J., de Cupeiro K., Jiménez N., Ruiz S., et al. . (2015). Scalable efficient expansion of mesenchymal stem cells in xeno free media using commercially available reagents. J. Transl. Med. 13, 232. 10.1186/s12967-015-0561-6 [PMC free article] [PubMed] [Cross Ref]
- Roberts I., Baila S., Rice R. B., Janssens M. E., Nguyen K., Moens N., et al. . (2012). Scale-up of human embryonic stem cell culture using a hollow fibre bioreactor. Biotechnol. Lett. 34, 2307–2315. 10.1007/s10529-012-1033-1 [PubMed] [Cross Ref]
- Rojewski M. T., Fekete N., Baila S., Nguyen K., Fürst D., Antwiler D., et al. . (2013). GMP-compliant isolation and expansion of bone marrow-derived MSCs in the closed, automated device quantum cell expansion system. Cell Transplant 22, 1981–2000. 10.3727/096368912X657990 [PubMed] [Cross Ref]
- Sajic M., Hunt D. P., Lee W., Compston D. A., Schweimer J. V., Gregson N. A., et al. . (2012). Mesenchymal stem cells lack efficacy in the treatment of experimental autoimmune neuritis despite in vitro inhibition of T-cell proliferation. PLoS ONE 7:e30708. 10.1371/journal.pone.0030708 [PMC free article] [PubMed] [Cross Ref]
- Sart S., Song L., Li Y. (2015). Controlling redox status for stem cell survival, expansion, and differentiation. Oxid. Med. Cell. Longev. 2015:105135. 10.1155/2015/105135 [PMC free article] [PubMed] [Cross Ref]
- Schneider N., Gonçalves Fda C., Pinto F. O., Lopez P. L., Araujo A. B., Pfaffenseller B., et al. . (2015). Dexamethasone and azathioprine promote cytoskeletal changes and affect mesenchymal stem cell migratory behavior. PLoS ONE 10:e0120538. 10.1371/journal.pone.0120538 [PMC free article] [PubMed] [Cross Ref]
- Schweizer R., Tsuji W., Gorantla V. S., Marra K. G., Rubin J. P., Plock J. A. (2015). The role of adipose-derived stem cells in breast cancer progression and metastasis. Stem Cells Int. 2015:120949. 10.1155/2015/120949 [PMC free article] [PubMed] [Cross Ref]
- Sdrimas K., Kourembanas S. (2014). MSC microvesicles for the treatment of lung disease: a new paradigm for cell-free therapy. Antioxid. Redox Signal. 21, 1905–1915. 10.1089/ars.2013.5784 [PMC free article] [PubMed] [Cross Ref]
- Semedo P., Correa-Costa M., Antonio Cenedeze M., Maria Avancini Costa Malheiros D., Antonia dos Reis M., Shimizu M. H., et al. . (2009). Mesenchymal stem cells attenuate renal fibrosis through immune modulation and remodeling properties in a rat remnant kidney model. Stem Cells 27, 3063–3073. 10.1002/stem.214 [PubMed] [Cross Ref]
- Sethe S., Scutt A., Stolzing A. (2006). Aging of mesenchymal stem cells. Ageing Res. Rev. 5, 91–116. 10.1016/j.arr.2005.10.001 [PubMed] [Cross Ref]
- Siegel G., Kluba T., Hermanutz-Klein U., Bieback K., Northoff H., Schäfer R. (2013). Phenotype, donor age and gender affect function of human bone marrow-derived mesenchymal stromal cells. BMC Med. 11:146. 10.1186/1741-7015-11-146 [PMC free article] [PubMed] [Cross Ref]
- Stern-Straeter J., Bonaterra G. A., Juritz S., Birk R., Goessler U. R., Bieback K., et al. . (2014). Evaluation of the effects of different culture media on the myogenic differentiation potential of adipose tissue- or bone marrow-derived human mesenchymal stem cells. Int. J. Mol. Med. 33, 160–170. 10.3892/ijmm.2013.1555 [PubMed] [Cross Ref]
- Stewart K., Walsh S., Screen J., Jefferiss C. M., Chainey J., Jordan G. R., et al. . (1999). Further characterization of cells expressing STRO-1 in cultures of adult human bone marrow stromal cells. J. Bone Miner. Res. 14, 1345–1356. 10.1359/jbmr.1999.14.8.1345 [PubMed] [Cross Ref]
- Stute N., Holtz K., Bubenheim M., Lange C., Blake F., Zander A. R. (2004). Autologous serum for isolation and expansion of human mesenchymal stem cells for clinical use. Exp. Hematol. 32, 1212–1225. 10.1016/j.exphem.2004.09.003 [PubMed] [Cross Ref]
- Succar P., Breen E. J., Kuah D., Herbert B. R. (2015). Alterations in the secretome of clinically relevant preparations of adipose-derived mesenchymal stem cells cocultured with hyaluronan. Stem Cells Int. 2015:421253. 10.1155/2015/421253 [PMC free article] [PubMed] [Cross Ref]
- Sung J. H., An H. S., Jeong J. H., Shin S., Song S. Y. (2015). Megestrol acetate increases the proliferation, migration, and adipogenic differentiation of adipose-derived stem cells via glucocorticoid receptor. Stem Cells Transl. Med. 4, 789–799. 10.5966/sctm.2015-0009 [PMC free article] [PubMed] [Cross Ref]
- Tajiri N., Duncan K., Borlongan M. C., Pabon M., Acosta S., de la Pena I., et al. . (2014). Adult stem cell transplantation: is gender a factor in stemness? Int. J. Mol. Sci. 15, 15225–15243. 10.3390/ijms150915225 [PMC free article] [PubMed] [Cross Ref]
- Tang L., Chen Y., Pei F., Zhang H. (2015). Lithium chloride modulates adipogenesis and osteogenesis of human bone marrow-derived mesenchymal stem cells. Cell. Physiol. Biochem. 37, 143–152. 10.1159/000430340 [PubMed] [Cross Ref]
- Tekkatte C., Gunasingh G. P., Cherian K. M., Sankaranarayanan K. (2011). “Humanized” stem cell culture techniques: the animal serum controversy. Stem Cells Int. 2011:504723. 10.4061/2011/504723 [PMC free article] [PubMed] [Cross Ref]
- Todeschi M. R., El Backly R., Capelli C., Daga A., Patrone E., Introna M., et al. . (2015). Transplanted umbilical cord mesenchymal stem cells modify the in vivo microenvironment enhancing angiogenesis and leading to bone regeneration. Stem Cells Dev. 24, 1570–1581. 10.1089/scd.2014.0490 [PMC free article] [PubMed] [Cross Ref]
- Tran C., Damaser M. S. (2015). Stem cells as drug delivery methods: application of stem cell secretome for regeneration. Adv. Drug Deliv. Rev. 82–83, 1–11. 10.1016/j.addr.2014.10.007 [PMC free article] [PubMed] [Cross Ref]
- Tsai T. L., Wang B., Squire M. W., Guo L. W., Li W. J. (2015). Endothelial cells direct human mesenchymal stem cells for osteo- and chondro-lineage differentiation through endothelin-1 and AKT signaling. Stem Cell Res. Ther. 6, 88. 10.1186/s13287-015-0065-6 [PMC free article] [PubMed] [Cross Ref]
- Tsuji W., Schnider J. T., McLaughlin M. M., Schweizer R., Zhang W., Solari M. G., et al. . (2015). Effects of immunosuppressive drugs on viability and susceptibility of adipose- and bone marrow-derived mesenchymal stem cells. Front. Immunol. 6:131. 10.3389/fimmu.2015.00131 [PMC free article] [PubMed] [Cross Ref]
- Vanden Berg-Foels W. S. (2014). In situ tissue regeneration: chemoattractants for endogenous stem cell recruitment. Tissue Eng. Part B Rev. 20, 28–39. 10.1089/ten.teb.2013.0100 [PMC free article] [PubMed] [Cross Ref]
- Wagner W., Bork S., Lepperdinger G., Joussen S., Ma N., Strunk D., et al. . (2010). How to track cellular aging of mesenchymal stromal cells? Aging (Albany NY) 2, 224–230. [PMC free article] [PubMed]
- Wagner W., Wein F., Seckinger A., Frankhauser M., Wirkner U., Krause U., et al. . (2005). Comparative characteristics of mesenchymal stem cells from human bone marrow, adipose tissue, and umbilical cord blood. Exp. Hematol. 33, 1402–1416. 10.1016/j.exphem.2005.07.003 [PubMed] [Cross Ref]
- Wang H., Gomez J. A., Klein S., Zhang Z., Seidler B., Yang Y., et al. . (2013a). Adult renal mesenchymal stem cell-like cells contribute to juxtaglomerular cell recruitment. J. Am. Soc. Nephrol. 24, 1263–1273. 10.1681/ASN.2012060596 [PMC free article] [PubMed] [Cross Ref]
- Wang J., Liao L., Wang S., Tan J. (2013b). Cell therapy with autologous mesenchymal stem cells-how the disease process impacts clinical considerations. Cytotherapy 15, 893–904. 10.1016/j.jcyt.2013.01.218 [PubMed] [Cross Ref]
- Wang X., Zhang H., Nie L., Xu L., Chen M., Ding Z. (2014). Myogenic differentiation and reparative activity of stromal cells derived from pericardial adipose in comparison to subcutaneous origin. Stem Cell Res. Ther. 5, 92. 10.1186/scrt481 [PMC free article] [PubMed] [Cross Ref]
- Wong S. P., Rowley J. E., Redpath A. N., Tilman J. D., Fellous T. G., Johnson J. R. (2015). Pericytes, mesenchymal stem cells and their contributions to tissue repair. Pharmacol. Ther. 151, 107–120. 10.1016/j.pharmthera.2015.03.006 [PubMed] [Cross Ref]
- Yavagal D. R., Lin B., Raval A. P., Garza P. S., Dong C., Zhao W., et al. . (2014). Efficacy and dose-dependent safety of intra-arterial delivery of mesenchymal stem cells in a rodent stroke model. PLoS ONE 9:e93735. 10.1371/journal.pone.0093735 [PMC free article] [PubMed] [Cross Ref]
- Yoon N., Park M. S., Peltier G. C., Lee R. H. (2015). Pre-activated human mesenchymal stromal cells in combination with doxorubicin synergistically enhance tumor-suppressive activity in mice. Cytotherapy 17, 1332–1341. 10.1016/j.jcyt.2015.06.009 [PubMed] [Cross Ref]
- Zanotti S., Kalajzic I., Aguila H. L., Canalis E. (2014). Sex and genetic factors determine osteoblastic differentiation potential of murine bone marrow stromal cells. PLoS ONE 9:e86757. 10.1371/journal.pone.0086757 [PMC free article] [PubMed] [Cross Ref]
- Zhang H., Fazel S., Tian H., Mickle D. A., Weisel R. D., Fujii T., et al. . (2005). Increasing donor age adversely impacts beneficial effects of bone marrow but not smooth muscle myocardial cell therapy. Am. J. Physiol. Heart Circ. Physiol. 289, H2089–H2096. 10.1152/ajpheart.00019.2005 [PubMed] [Cross Ref]
- Zhao H., Feng J., Ho T. V., Grimes W., Urata M., Chai Y. (2015). The suture provides a niche for mesenchymal stem cells of craniofacial bones. Nat. Cell Biol. 17, 386–396. 10.1038/ncb3139 [PMC free article] [PubMed] [Cross Ref]