Abstract
Previously, we reported that a novel subpopulation of young mesenchymal stem cells (YMSCs) existed in old bone marrow, which possessed high antiaging properties as well as excellent efficacy for cardiac repair. MicroRNAs (miRNAs) have emerged as key regulators in post-transcriptional gene expression programs, and however, it is unknown whether miRNAs directly control stem cell senescence. Here we present the first evidence that miR-195 overexpressed in old MSCs (OMSCs) induces stem cell senescence deteriorating their regenerative ability by directly deactivating telomerase reverse transcriptase (Tert), and abrogation of miR-195 can reverse stem cell aging. MiRNAs profiling analysis in YMSCs and OMSCs by microarray showed that miR-140, miR-146a/b, and miR-195 were significantly upregulated in OMSCs, which led us to hypothesize that these are age-induced miRNAs involved in stem cell senescence. Of these miRNAs, we found miR-195 directly targeted 3′-untranslated region of Tert gene by computational target prediction analysis and luciferase assay, and knockdown of miR-195 significantly increased Tert expression in OMSCs. Strikingly, miR-195 inhibition significantly induced telomere relengthening in OMSCs along with reduced expression of senescence-associated β-galactosidase. Moreover, silencing miR-195 in OMSCs by transfection of miR-195 inhibitor significantly restored antiaging factors expression including Tert and Sirt1 as well as phosphorylation of Akt and FOXO1. Notably, abrogation of miR-195 markedly restored proliferative abilities in OMSCs. Transplantation of OMSCs with knocked out miR-195 reduced infarction size and improved LV function. In conclusion, rejuvenation of aged stem cells by miR-195 inhibition would be a promising autologous therapeutic strategy for cardiac repair in the elderly patients. Stem Cells 2016;34:148–159
Significance Statement
In this study, we investigated the role of miRNAs involved in stem cell aging and their impact on cardiac repair. Here we report our novel findings that regenerative ability of aged stem cells is deteriorated by increased expression of miR-195 which targets telomerase reverse transcriptase (Tert) gene, and abrogation of miR-195 in aged stem cells markedly induces Tert reactivation, resulting in significant telomere re-lengthening. Additionally, we show that transplantation of aged stem cells lacking miR-195 significantly reduced infarction size and concomitant improvement of cardiac function. These findings demonstrate that silencing miR-195 reverses the senescence clock in aged stem cells by telomerase reactivation. The strategy of modification of miRs in stem cells holds great promise for stem cell based repair of aging myocardium.
Introduction
Stem cells regenerate multiple adult tissues including heart, brain, nerves, blood, skin, and skeletal muscle [1]. There is a decline in tissue regenerative ability with aging. Age-induced alterations in the function of stem cells may impair regeneration in age-related diseases [2]. However, it remains largely unknown whether a decline in stem cell function with aging influences mammalian life span. The most significant phenotype in mammalian aging is telomere shortening which induces disruption of chromosome integrity by DNA damage and apoptosis [3]. Telomerase lengthens telomeres in DNA strands by adding DNA sequence repeats (“TTAGGG” in all vertebrates) to the 3′ end of DNA strands in the telomere regions, which are found at the ends of eukaryotic chromosomes, thereby preventing senescent cells from postmitotic condition and apoptosis [4]. However, telomerase activity tends to decline with aging and loss of telomerase function in mice reduces the regenerative capacity of proliferative organs, accelerates the development of aging phenotypes, reduces life span, and increases cancer incidence [5]. Telomerase-deficient mice exhibit defects in stem cell function in the forebrain, epidermis, intestinal epithelium, and hematopoietic system [6]. Telomerase knockout mice suffer from severe left ventricular (LV) failure [7] and telomerase expression and activity are also markedly decreased in the adult heart [8]. Patients with chronic heart failure also have 25% shorter cardiac telomeres compared to healthy age controls [9], Furthermore, shorter cardiac telomeres were found in aged hearts with mild hypertrophy and biventricular failure [10], suggesting that telomere length is associated with the severity of heart failure.
Bone marrow stem cells (BMSCs) are heterogeneous population including small cells and large cells, and generally, BMSC population in aged animals contains more senescent large cells than that from young animals [11]. Previously, Kucia et al. isolated a population of rare (∼0.01%) Sca-1+/Lin−/CD45− cells from bone marrow mononuclear cells (termed as very small embryonic like stem cells; VSELs) [12]. VSELs express pluripotency markers, SSEA-1, Oct-4, Nanog, and Rex-1 as well as telomerase protein Rif-1. Recently, we have also discovered that a subpopulation of small young mesenchymal stem cells (YMSCs) exists in the old BMSCs [13]. These cells highly express antiaging genes, pluripotency genes, and cardiac differentiation markers. Furthermore, transplantation of YMSCs into the infarcted murine heart exhibited increased neovascularization and cardiomyogenesis and improved cardiac functions, as compared to OMSCs. Although it remained unclear whether or not these small cells are pluripotent stem cells, the juvenile subpopulation of MSCs which exists in the aged bone marrow possesses antiaging properties of YMSCs and can be exploited for stem cell-based therapy in elderly patients.
MicroRNAs (miRNAs) have emerged as key regulators in the gene expression program associated with cellular senescence [14]. Hackl et al. have reported that miR-17 family was downregulated in aged tissues and cells [15], and another study by Faraonio et al. showed that replicative senescence in normal human diploid fibroblasts is accompanied by altered expression of a set of miRNAs, with 14 and 10 miRNAs being either upregulated or downregulated [16]. These studies suggest that some miRNAs contribute to cellular senescence at least in part. However, it is unclear whether changes in miRNA profiles are directly involved in the onset of pathophysiological changes or rather outcome of the senescence process resulting from tissue aging and dysfunction.
In this study, we investigated the role of miRNAs involved in stem cell aging and their impact on cardiac repair. Here we report our novel findings that regenerative ability of aged stem cells are deteriorated by increased expression of miR-195 which targets telomerase reverse transcriptase (Tert) gene, and abrogation of miR-195 in aged stem cells markedly induces Tert reactivation, resulting in significant telomere relengthening. Additionally, we show that transplantation of aged stem cells lacking miR-195 significantly reduced infarction size and concomitant improvement of cardiac function. These findings demonstrate that silencing miR-195 reverses the senescence clock in aged stem cells by telomerase reactivation and this strategy holds a great promise for stem cell based repair of aging myocardium.
Materials and Methods
Isolation of OMSCs and YMSCs from Bone Marrow
Our study followed the Guide for the Care and Use of Laboratory Animals published by the US National Institutes of Health (NIH Publication #85-23, revised 1985) and protocol approved by the Institutional Animal Care and Use Committee, University of Cincinnati. BMSCs were isolated from bone marrow of C57BL/6 mice (young; 2 months, old; 24 months, respectively) as described previously [13]. Briefly, BMSCs were cultured in 100 mm dishes with low glucose Dulbecco's modified Eagle's medium (DMEM) (HyClone Laboratories, Logan, UT, http://www.hyclone.com) supplemented with 10% fetal bovine serum (FBS) for 6–10 days. The nonadherent hematopoietic bone marrow cells were discarded during the routine fresh medium replacement. YMSCs were isolated from old bone marrow by using 3 μm pores (cell culture insert; BD Bioscience, San Diego, CA, http://www.bdbiosciences.com). The homogenicity of YMSC population was confirmed with surface marker expression by fluorescence activated cell sorting analysis as described previously [13].
Quantitative Fluorescence In Situ Hybridization for Telomere Length Measurement
Telomere was detected by fluorescence in situ hybridization (FISH) with PNA Telomere Cy3-labbeled probe (F1002; TelC-Cy3, PNA Bio) according to manufacturer's instructions. Briefly, cells were fixed with 4% paraformaldehyde for 1 hour. After washing with phosphate buffer solution (PBS), cells were incubated with RNase 100 ng/μL for 20 minutes and 0.005% pepsin for 5 minutes at 37 °C. After dehydration with 70%, 85%, and 100% of cold ethanol, cells were incubated with 200 nM PNA Telomere probe for 10 minutes at 85 °C and incubated for 2 hours at room temperature. Then, cells were incubated with 2 × saline-sodium citrate (SSC) buffer for 10 minutes at 60 °C. 4',6-diamidino-2-phenylindole (DAPI) and Cy3 signals were acquired simultaneously into separate channels using a confocal microscope (Fluoview FV1000, Olympus, Tokyo, http://www.olympus-global.com), and maximum projection from image stacks (10 sections at steps of 1 µm) were generated for image quantification. Telomere length was analyzed by using TFL-TeloV2-2 free software (Vancouver, Canada). With the program, the integrated fluorescence intensity value for each telomere, which is proportional to the number of hybridized probes, is calculated and presented. TFL-Telo is an application program used to estimate the length of telomeres from captured images of metaphases that have been stained for quantitative FISH (Q-FISH) analysis [17].
Reverse Transcription-Polymerase Chain Reaction Analysis
Total RNA was isolated from various treatment groups of the cells with RNeasy Mini Kit (Qiagen, MD, http://www1.qiagen.com), and cDNA was prepared using Omniscript-RT Kit (Qiagen), according to the manufacturer's instructions. For polymerase chain reaction (PCR) amplification, 1 μg of the cDNA from the reverse transcription reaction was then added to a PCR mix containing the suggested quantity of Qiagen PCR buffer, Q-Solution, dNTP mix, reverse and forward primers, Taq DNA polymerase, and distilled water. Each PCR reaction was performed with specific primers.
Isolation and Detection of miRNA
Extraction of miRNAs was performed by using mirVana miRNA Isolation Kit, and miR-195 expression was detected by using mirVana qRT-PCR miRNA Detection Kit (Ambion, Life Technologies, Austin, TX, http://www.ambion.com) and QuantiTect SYBR green PCR kit (Qiagen) as previously described [18]. Specific miRNA primers were purchased from Ambion.
miRNA Microarray
Total RNA samples obtained from OMSCs and YMSCs were sent to Exiqon (Denmark, http://www.exiqon.com/) for miRNA microarray profiling. Data were analyzed by Exiqon with in-house developed computer programs. Intensity values were transformed into log2 scale, and fold changes were given in log2 scale. A t test was performed between OMSCs and YMSCs profiling, and statistically significant difference was considered at p < .01.
FISH to Detect miR-195
In situ detection of miR-195 was performed in OMSCs and YMSCs plated on chamber slide. Samples were fixed in 4% paraformaldehyde for cell culture at room temperature for 20 minutes followed by two washes in PBS. Fixed cells were then prehybridized in hybridization solution (BioChain, CA) for 3 hours at room temperature before hybridization. Probe (3 pmol; LNA-modified and fluorescein isothiocyanate (FITC)-labeled oligonucleotide; purchased from Exiqon) complementary to miR-195 was hybridized to the cells for 13–16 hours at 22 °C lower than predicted Tm of the probe. Subsequent to posthybridization washes with SSC buffer, in situ hybridization signals were detected with confocal microscope (Fluoview FV1000, Olympus).
Luciferase Activity Assay
Precursor miR-195 expression vector was constructed in a feline immunodeficiency virus-based lentiviral vector system (Genecopoeia). Luciferase reporter constructs containing 3′-untranslational region (UTR) of mouse Tert was designed to encompass mmu-miR-195 binding sites. Cells were plated into 24-well plates in triplicate and cotransfected with miR-195 vector (or scramble vector) and reporter construct with Lipofectamine 2000 (Invitrogen, Carlsbad, CA, http://www.invitrogen.com). Firefly luciferase activities were measured by using Dual Luciferase Reporter Assay System kit (Promega, Madison, WI, http://www.promega.com) per manufacturer's instructions. Transfection efficiency was normalized by Renilla luciferase activity.
Lentivirus-Mediated miR-195 Inhibition in OMSCs
Lentivirus containing miR-195 inhibitor-expressing vector was generated by using Lenti-Pac HIV Expression Packaging System (GeneCopoeia) per manufacturer's protocol. Briefly, 2.5 µL of lentiviral miR-195 inhibitor expression plasmid or scramble, 5.0 µL of EndoFectin Lenti and EndoFectin Lenti reagent were added in Opti-MEM I, and formed the DNA-EndoFectine complex. After incubating the complex at room temperature for 10–25 minutes, the DNA–EndoFectine complex was added to 293Ta cells in DMEM with 10% FBS, and then, incubated in 5% CO2 at 37 °C overnight. The culture medium was replaced with fresh DMEM with 5% FBS and 1/500 volume of the TiterBoost reagent to the culture medium. The virus pseudovirus-containing culture medium was collected at 48 hours post-transfection and concentrated after filtration. For the transduction of OMSCs with lentivirus, 10 × 106 of OMSCs was plated, and 20 µL of virus suspension was added. To enhance lentiviral transduction efficiency, cells were placed at 4 °C for 2 hours and then incubated in a 5% CO2 at 37 °C for 48 hours.
Senescence-Associated β-Galactosidase (gal) Staining
Senescence-associated β-gal was detected by Senescence Detection Kit (BioVision, CA, http://www.biovision.com/) per manufacturer's instruction.
Terminal Deoxynucleotidyl Transferase dUTP Nick End Labeling Assay
Apoptotic cell death was detected by terminal deoxynucleotidyl transferase dUTP nick end labeling (TUNEL) according to instructions of the manufacturer (TMR Red; Roche Applied Science, http://www.roche-applied-science.com). For quantification, the numbers of TUNEL positive cells were counted in at least five randomly selected high-power fields (magnification × 200) with three independent samples.
Western Blot Analysis
Western blot was carried out as previously described [19]. Briefly, cells were lysed in lysis buffer, pH 7.4 [(in mM) 50 HEPES, 5 EDTA, 50 NaCl], 1% Triton X-100, protease inhibitors [(10 µg/mL aprotinin, 1 mM phenylmethylsulfonyl fluoride, 10 µg/mL leupeptin) and phosphatase inhibitors [(in mM) 50 sodium fluoride, 1 sodium orthovanadate, 10 sodium pyrophosphate]. The protein samples (40 µg) were electrophoresed using SDS-polyacrylamide gel and electroimmunoblotted. The specific antibodies used for the detection of Tert (sc-68720) was purchased from Santa Cruz (Santa Cruz, CA, http://www.scbt.com), p53 (#2524), Sirt-1 (#2028), Phospho-FoxO1 (#2599), Bcl-2 (#3498), cleaved caspase-3 (#9661), Akt (#2920), Phospho Akt (#4060), and Actin (#4968) were purchased from Cell Signaling (Beverly, MA, http://www.cellsignal.com). All antibodies were used as diluted with 1:1,000.
Determination of Cell Proliferation Rate
Cell proliferation rate was determined by cell growth assay and colony formation assay as previously reported [13].
Experimental Model of Acute Myocardial Infarction and Cell Transplantation
In vivo cell transplantation using mouse acute myocardial infarction (AMI) model was performed in accordance with our previous report [20]. Briefly, C57BL/6 mice (male 24 months old, 30–35 g b. wt.; n = 10 animals per group) were prepared for left anterior descending (LAD) coronary artery ligation. Intraperitoneal anesthesia was administered with 0.1% of ketamine and 0.02% of xylene per body weight (g) for anesthesia. After endotracheal intubation and mechanical ventilation using Rodent Ventilator (Harvard Apparatus Model 683), the heart was exposed by left-sided minimal thoracotomy and LAD coronary artery was ligated with 6-0 silk. Immediately after ligation, 20 μL DMEM without cells or containing 2 × 105 cells (scrOMSCs or anti-195OMSCs) were injected into infarction and border zones. Following injection, the opened chests of mice were sutured and all mice were allowed to recover.
Physiologic Assessment of Heart Function
Transthoracic echocardiography was performed to study change in the heart function at 4 weeks after transplantation by using iE33 equipped with 15 to 7 MHz broadband transducer (Philips Ultrasound, Bothell, WA) as previously described [20]. Heart was imaged in the two-dimensional mode in the parasternal long-axis and/or parasternal short-axis views which were subsequently used to position the M-mode cursor perpendicular to the ventricular septum and LV posterior wall, after which M-mode images were obtained. Measurements of ventricular anterior thickness, LV internal dimension, and LV posterior wall thickness were made from two-dimensionally directed M-mode images of the LV in both systole and diastole. Ejection fraction (EF) was measured based on area-length method after measurement of LV internal area and distance from apex to basal LV in both systole and diastole. All the scoring system was patterned after the American Society of Echocardiography's scoring system used conventionally in interpreting clinical echocardiographic studies.
Infarction Size Determination
For measurement of infarction size and area of fibrosis, the hearts were arrested in diastole by intravenous injection of cadmium chloride and fixed in 10% buffered formalin. The heart was excised, cut transversely, and embedded in paraffin for Masson's trichrome staining. Infarct size was defined as sum of the epicardial and endocardial infarct circumference divided by sum of the total LV epicardial and endocardial circumferences using computer based planimetry with Image-J analysis software (version 1.6; NIH).
Statistical Analysis
All values were expressed as mean ± SE. Comparison between two mean values was evaluated by an unpaired Student's two-tailed t test and between three or more groups was evaluated by one-way ANOVA followed by Bonferroni post hoc analysis. Statistical significance was accepted at p < .05.
Results
OMSCs Exhibit the Shorter Telomeres Along with Impaired Expression of Antiaging Genes
We have previously reported that both YMSCs and OMSCs are positive for MSC markers, CD29, CD44, and CD90, but negative for CD45 and CD119. We also showed that expression of pluripotency markers including Oct-4, Nanog, Sox-2, and Klf-4 was significantly upregulated in YMSCs as compared to OMSCs [13]. To validate our previous data that OMSCs expressed characteristics specific for senescence, we compared telomere length as well as antiaging genes expression between OMSCs and YMSCs. As compared to YMSCs, OMSCs showed markedly short telomeres as examined by Q-FISH analysis (Fig. 1A). In addition, we observed that average nucleus size of OMSCs was bigger than that of YMSCs (12 µm vs. 5 µm). Expressions of representative antiaging genes including Sirt1, Tert and antiapoptotic genes Bcl-2 were markedly reduced in OMSCs as compared to YMSCs (Fig. 1B). These results are in agreement with our previous report that OMSCs exhibited the impaired expression of cardiac lineage markers (Gata-4 and Mef2C) and pluripotency markers (Oct-4, Sox-2, SSEA-1, and Nanog) as well as senescence-associated β-gal expression, as compared to YMSCs [13].
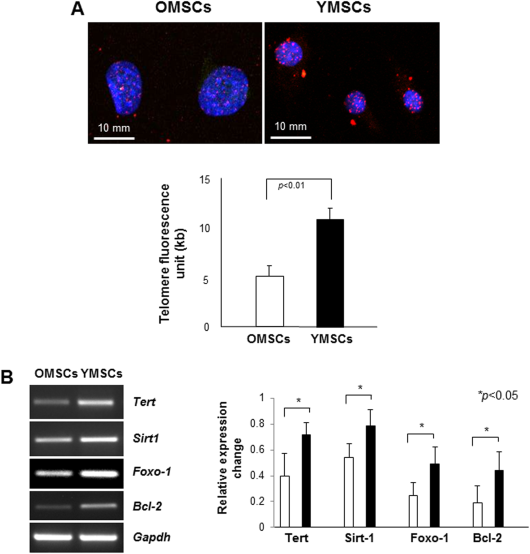
Figure 1. OMSCs exhibit the shorter telomeres with impaired expression of antiaging genes. (A): Quantitative fluorescence in situ hybridization analysis showed that OMSCs have shorter telomeres as compared to YMSCs. (B): Expressions of antiaging genes (Tert, Sirt1) and antiapoptotic gene (Bcl2) were impaired in OMSCs when compared to YMSCs. Abbreviations: OMSCs, old mesenchymal stem cells; Tert, telomerase reverse transcriptase; YMSCs, young mesenchymal stem cells.
miR-195 Expression Is Markedly Upregulated in OMSCs
To profile miRNA expression induced with aging, we isolated total RNA from OMSCs and YMSCs, and performed microarray analysis in OMSCs and YMSCs (Fig. 2A). Per our results, the expression of miR-140, miR-146a/b, and miR-195 was significantly upregulated in OMSCs whereas expression of miR-29b, miR-205, miR-378, and miR-542-3p was downregulated in OMSCs (Fig. 2B, 2C). Reverse transcriptase PCR (RT-PCR) and real time PCR confirmed microarray result that miR-195 expression was significantly upregulated in OMSCs as compared to YMSCs (Fig. 2D, 2E). FISH analysis for miR-195 visualization further confirmed that miR-195 was highly expressed in OMSCs (Fig. 2F). This result led us to hypothesize that miR-195 was induced with aging, and its abrogation in OMSCs may restore the regenerative capacity of OMSCs. We transfected OMSCs with anti-miR-195 and confirmed that transfected OMSCs showed reduced expression of miR-195 while scramble did not affect it, as shown by real-time RT-PCR (Fig. 2G), indicating that miR-195 inhibitor we used was specific for reduction of miR-195 expression in stem cells.
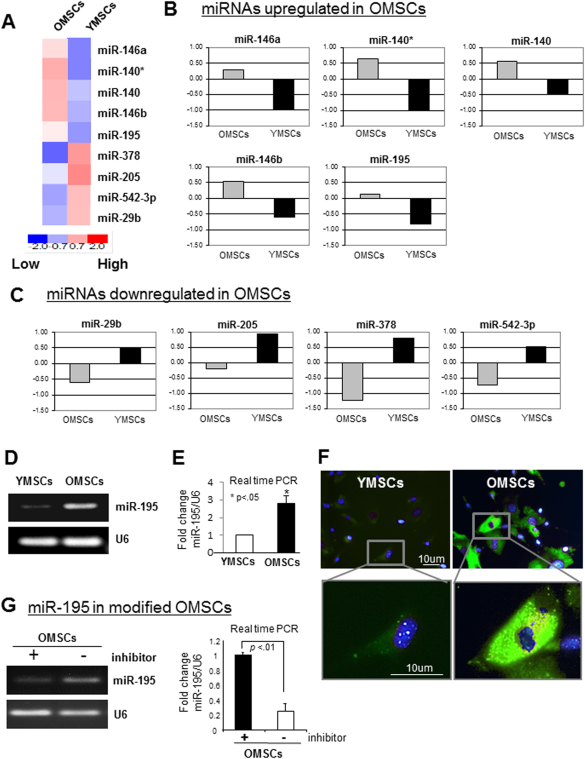
Figure 2. miR-195 expression is highly upregulated in OMSCs. (A–C): miRNA microarray analysis was performed to profile miRNAs expression in OMSCs and YMSCs. Expression of miR-140, 146a/b, and 195 was significantly upregulated in OMSCs while miR-29b, miR-205, miR-378, and miR-542-3p were downregulated. (D, E): Higher expression of miR-195 in OMSCs was confirmed by reverse transcriptase PCR. (F): Fluorescence in situ hybridization (FISH) analysis also indicated higher expression of miR-195 in OMSCs as compared to YMSCs. FISH was performed by using miR-195 specific 5′-fluorescein labeled miRCURY LNA detection probe. (G): The expression of miR-195 in OMSCs transfected with anti-miR-195 and that transfected with scramble miR. Abbreviations: miRNAs, microRNAs; OMSCs, old mesenchymal stem cells; PCR, polymerase chain reaction; YMSCs, young mesenchymal stem cells.
Tert Is a Direct Target of miR-195 in Aging Stem Cells
To investigate the biological relevance of miR-195 induction during stem cell aging and its participation in OMSCs senescence, we first carried out target prediction analysis by in silico search to find potential target genes of miR-195 responsible for aging and senescence. Interestingly, computational analysis predicted that mmu-miR-195 directly binds to 3′-UTR of mouse Tert gene (Fig. 3A). To experimentally validate this result, we performed RT-PCR and Western blot analysis to examine whether Tert expression is altered by miR-195 knockdown in OMSCs. Interestingly, expression of both mRNA and protein of Tert was significantly increased by miR-195 abrogation in OMSCs (Fig. 3B, 3C), indicating that Tert is a putative target of miR-195. Tert as a target gene of miR-195 was confirmed by luciferase activity assay which showed that cotransfection of a miR-195 expression vector (pEZX-miR-195) with the vector containing 3′-UTR of Tert gene significantly reduced luciferase activity in comparison to cotransfection with miR-scramble vector (pEZX-miR-Sc) (Fig. 3D). These results demonstrated that Tert is a direct target of miR-195 in stem cell aging.
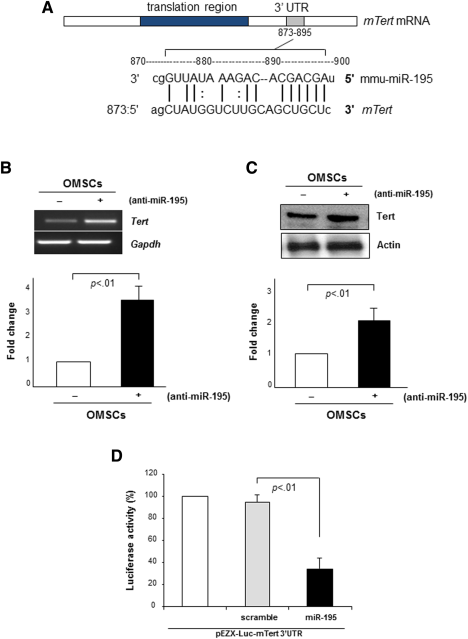
Figure 3. Tert is a direct target of miR-195 in stem cell aging. (A): Computational analysis predicted mTert as a potential target of mmu-miR-195. (B, C): Transfection of OMSCs with anti-miR-195 restored expression of Tert mRNA as well as telomere specific protein TRF2, as examined by reverse transcriptase polymerase chain reaction and Western blot, respectively. (D): Cotransfection with pEZX-Luc vector containing mTert 3′ UTR and a plasmid encoding miR-195 showed decreased luciferase activity (p < .01 vs. pEZX-miR-SC transfected cells). Transfection efficiency was normalized by Renilla luciferase activity. Abbreviations: OMSCs, old mesenchymal stem cells; Tert, telomerase reverse transcriptase; UTR, untranslated region.
Abrogation of miR-195 Rejuvenates OMSCs Through Telomere Relengthening and Antiaging Markers Reactivation
To examine the mechanistic participation of miR-195 in stem cell aging, we knocked down miR-195 expression by using a lentiviral miR-195 inhibitor vector (Lenti-anti-miR-195) which could successfully transfect OMSCs to achieve abrogation of miR-195 (Fig. 4A). mCherry signal (red fluorescence) in this vector system allowed us to recognize the transfected OSMCs with miR-195 inhibitor or scramble vector (Fig. 4B, upper panel). Interestingly, abrogation of miR-195 significantly reduced senescence-associated β-gal expression in OSMCs as compared to scramble transfected OMSCs (Fig. 4B, 20.6% ± 4.9% vs. 54.5% ± 8.1%, p < .01). More importantly, OMSCs transfected with miR-195 inhibitor induced significant telomere relengthening (2.9-fold higher as compared to scramble transfection, Fig. 4C). Furthermore, inhibition of miR-195 reduced TUNEL-positive apoptotic cell death in OMSCs (Fig. 4D, 19.7% ± 4.5% vs. 60.8% ± 2.7%, p < .01), suggesting that rejuvenated OMSCs by miR-195 abrogation survive better under apoptotic condition such as ischemia. Furthermore, expression of antiaging makers (Tert and Sirt-1) and prosurvival markers (p-Akt and Bcl-2) were significantly increased by transfection of anti-miR-195 in OMSCs whereas expression of senescence-associated markers (p53) and proapoptotic marker (cleaved caspase-3) was markedly reduced by miR-195 abrogation (Fig. 4E), supporting our hypothesis that inhibition of age-induced miR-195 can rejuvenate OMSCs by reactivation of antiaging factors and suppression of senescence-associated markers. It is important to note that knockdown of miR-195 significantly restored the impaired cell proliferative abilities in OMSCs as evaluated by cell proliferation assay and colony formation assay (Fig. 4F, 4G). These results demonstrated that miR-195 induced during stem cell aging plays critical roles in their fate and behaviors.
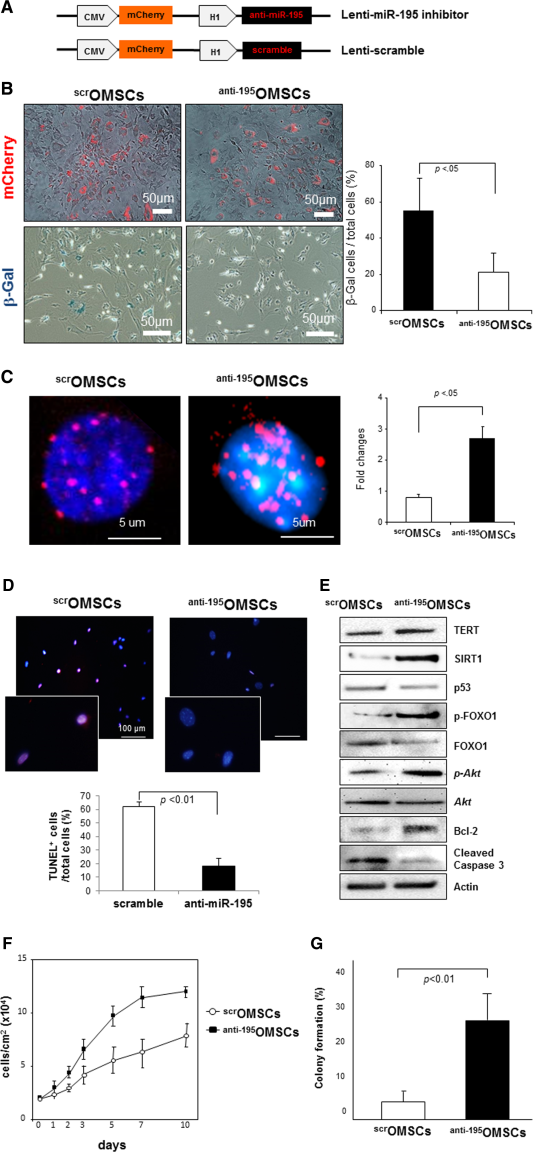
Figure 4. Abrogation of miR-195 rejuvenates OMSCs by telomere relengthening and antiaging markers reactivation. (A): Structures of Lenti-miR-195 inhibitor and Lenti-scramble which contain mCherry reporter gene (red signal). (B): Abrogation of miR-195 significantly reduced senescence-associated β-gal expression in OSMCs as compared to scramble transfected OMSCs. (C): OMSCs showed significant telomere relengthening when they were transfected with miR-195 inhibitor. (D): miR-195 inhibition markedly reduced terminal deoxynucleotidyl transferase dUTP nick end labeling-positive apoptotic cell death in OMSCs. (E): Expressions of TRF2, SIRT1, and Bcl-2 as well as phosphorylation of FOXO1 and Akt were significantly increased by transfection of anti-miR-195 in OMSCs whereas expression of p53 and cleaved caspase 3 was reduced by miR-195 abrogation. (F, G): Knockdown of miR-195 significantly restored cell proliferative abilities in OMSCs as examined by cell proliferation assay and colony formation assay. Abbreviation: OMSCs, old mesenchymal stem cells.
Transplantation of OMSCs Deleting miR-195 Shows the Improved Efficacy for Cardiac Repair
To investigate functional consequence and therapeutic relevance of age-induced miR-195 in ischemic heart repair, we transfected OMSCs with miR-195 inhibitor (anti-195OMSCs) or scramble (scrOMSCs), and then, transplanted into the infarcted mouse heart. All animals survived full length of studies and there were no deaths related to cell transplantation. The animals (n = 10 per group) were harvested at 4 weeks after respective treatment. Notably, transplantation of anti-195OMSCs significantly reduced infarction size with better efficacy when compared to that of scrOMSCs (anti-195OMSCs: 23.6% ± 10.2%, scrOMSCs: 41.8% ± 13.1%, p < .05) (Fig. 5A). To further investigate whether transplantation of anti-195OMSCs improves the therapeutic potential of aged stem cells for cardiac functions, LV function was assessed by echocardiography after cell transplantation. The indices of LV contractile function were well-preserved in anti-195OMSCs injection group compared to scrOMSCs injection group (Fig. 5B–5E). Indices of global heart functions were well preserved in anti-195OMSCs transplanted groups including LV diastolic volume (scrOMSCs: 132.7 ± 37.1 mm3, anti-195OMSCs: 94.5 ± 17.2 mm3, p < .05), LVEF (scrOMSCs: 28.5 ± 12.2%, anti-195OMSCs: 46.2% ± 12.7%, p < .05) and LV fraction shorting (LVFS) (scrOMSCs: 12.9% ± 3.5%, anti-195OMSCs: 24.8% ± 4.6%, p < .05). We also analyzed LV functions by TEI (myocardial performance index, Fig. 5D) and E/E′ (strong predictor of LV endiastolic developed pressure (LVEDP), Fig. 5E) since TEI is useful index for assessing cardiac systolic and diastolic function, and E/E′ is well-correlated with the prognosis of heart failure. TEI and E/E′ also indicated that anti-195OMSCs has better efficacy to improve cardiac functions as compared to scrOMSCs. Taken together, these results demonstrated that impaired regenerative abilities of the senescent stem cells can be remarkably reversed by abrogation of age-induced miR-195.
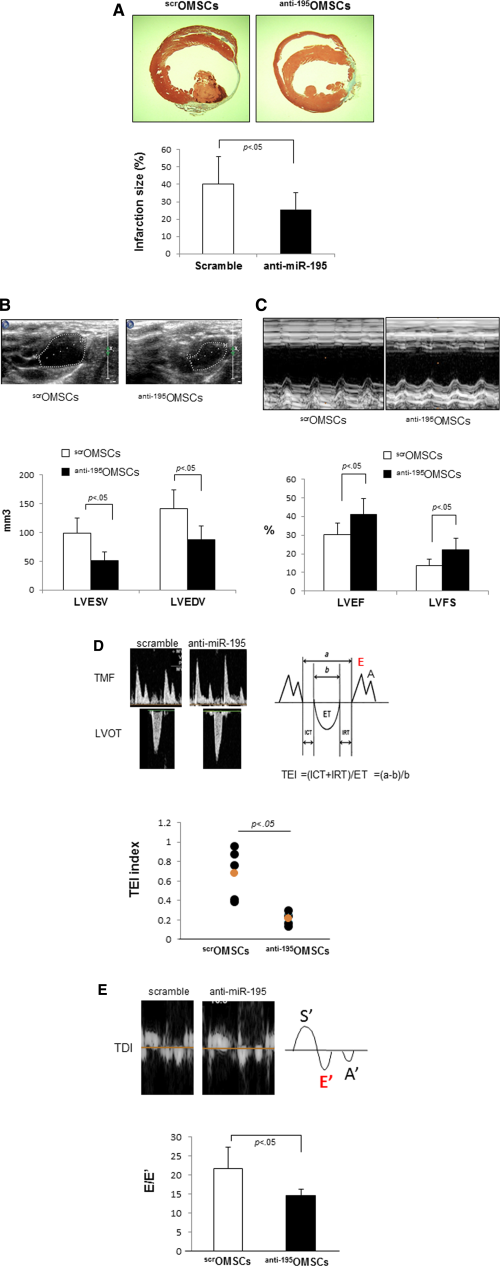
Figure 5. Transplantation of miR-195 deleted OMSCs shows the improved efficacy for cardiac repair. (A): Transplantation of OMSCs lacking miR-195 into the infarcted heart significantly reduced infarction size as determined by Masson trichrome staining and Image-J analysis software. Transplantation of OMSCs transfected with anti-miR-195 improved systolic functions (LVEDV, LVESV, LVEF, and LVFS) of the infarcted heart. (B): Representative figure of LV long axis view. LVESV and LVEDV. (C): Representative figure of LV M-mode image. LVEF and LVFS. Transplantation of OMSCs transfected with anti-miR-195 improved diastolic (TEI index and E/E′) functions of the infarcted heart. (D): TEI index; ICT+IRT/ET, myocardial performance index. (E): E/E′; peak velocity of early diastole mitral inflow, E divided peak velocity of mitral annulus measure by tissue Doppler, E′. Abbreviations: ET, ejection time; ICT, isovolumic contraction time; IRT, isovolumic relaxation time; LVESV; left ventricular endo systolic volume, LVEDV; left ventricular endo diastolic volume; LVEF; left ventricular ejection fraction; LVFS; left ventricular fractional shortening; Left ventricular outflow tract (LVOT), OMSCs, old mesenchymal stem cells; TDI, Tissue doppler image; TEI, the doppler total ejection isovolumic index; TMF, trans-mitral flow velocity pattern.
Discussion
Elderly patients are more likely to have cardiac arrest following MI [21]. Myocardial ischemia results in massive death of cardiomyocytes, and acceleration of cell death in the aged myocardium has been proposed as a mechanism for increased risk of heart failure in the elderly. Tissue-resident adult stem cells including cardiac stem cells maintain normal tissue homeostasis and participate in tissue repair and regeneration in response to damage [22]. Aging is accompanied by a decline in regenerative capacity including myocardial regeneration, wound healing, skeletal muscle mass, and strength and neurogenesis [23]. Since the regenerative activities of the damaged tissues are attributable to the resident adult stem cells, these age-related deterioration of organ functions may be due to the impaired functionality of stem cells [2, 24]. Therefore, it is important to understand the relationship between stem cell function and their ability to treat age-related degenerative diseases including cardiovascular diseases.
The main hypothesis of this study was that rejuvenation of the senescent stem cells with antiaging miR can improve their repair capacity of the aging myocardium following MI. Transplantation of rejuvenated OMSCs resulted in significant improvement of cardiac function and reduction of infarct size. The effects of MSCs could be through their direct differentiation into cells of cardiac lineage alone or together with paracrine effects. Previously, we reported that a novel subpopulation of YMSCs with high proliferative and differentiation capacity existed in the aged bone marrow, and transplantation of YMSCs into the infarcted heart was more effective to reduce the infarction size and to improve LV functions than OMSCs transplantation [13]. These findings led us to search for the key factors responsible for progression of stem cell aging and to hypothesize that maintaining juvenile properties, for example, antiaging, pluripotency, high proliferation and differentiation rates, would offer a great therapeutic strategy for repair of aging myocardium.
The major findings of this study are that (a) miR-195 expression is highly upregulated in OMSCs, (b) miR-195 directly targets 3′-UTR of Tert gene, thereby promoting telomere shortening in OMSCs, (c) abrogation of age-induced miR-195 rejuvenates OMSCs by reactivating Tert as well as antiaging factors, (d) genetic deletion of miR-195 in OMSCs can reverse their impaired regenerative abilities for cardiac repair. In the present study, we focused on age-induced miRNAs, especially for miR-195 to improve cell functional capacity of aging stem cells since miRNAs are key regulators in the gene expression program associated with cellular senescence [14]. Several studies have reported that miR-17 family was downregulated in aged tissues and cells in human [15], and senescence in human lung fibroblasts was accompanied by upregulation of miR-152, miR-410, miR-431, and miR-493, and downregulation of miR-155, miR-20a, miR-25, and miR-15a [25]. Another study showed that replicative senescence in normal human diploid fibroblasts is accompanied by altered expression of miRNAs [16]. Recently, Boon and Dimmeler et al. have reported that expression of age-induced miR-34a, targeting PNUT, is a key mechanism that regulates cardiac contractile function during aging and after AMI [26]. MiR-34a inhibition reduced telomere shortening, DNA damage and cardiomyocyte apoptosis, and improved functional recovery after MI. Taken together, these studies suggested that altered expression of miRNAs with aging is a key factor in organ dysfunctions, and modulation of age-induced miRNAs can reduce the effects of cellular senescence. In this study, we demonstrated for the first time that miR-195 expression was significantly induced with aging in bone marrow-derived stem cells and abrogation of age-induced miR-195 can rejuvenate the senescent MSCs by inducing telomerase reactivation, resulting in telomere relengthening. In addition, rejuvenation of OMSCs by knockdown of miR-195 resulted in increased cell proliferation and colony formation.
One of the important mechanisms for cellular senescence is downregulation of telomerase and the resulting loss of its activity. Telomere shortening induces disruption of chromosome integrity because uncapped telomeres activate signaling pathways associated with DNA damage and apoptosis [3]. Human adult hematopoietic and nonhematopoietic stem cells (HSCs) showed a decline in telomerase activity as well as immune response with aging [27, 28], and this could be correlated with telomere shortening in T- and B-lymphocytes [29, 30]. Cardiac stem cells (CSCs) play important roles in delaying cardiac aging and dysfunction through differentiation into cardiomyocytes, and lack of telomerase activity and short telomeres can induce CSCs senescence. Similarly, our previous study showed that MSCs obtained from the aged mice (20–24 months old) showed the impaired telomerase expression along with shorter telomeres, resulting in the impaired proliferation rate and differentiation ability as compared to that from young animals (2 months old) [31]. Other studies also showed that overexpression of telomerase in human MSCs increases proliferation and differentiation capacity [32], and the telomerase knockout MSCs did not differentiate into adipocytes or chondrocytes even at earlier passages [33]. Therefore, it is important to upregulate telomerase in the aged stem cells to promote regenerative potential as well as longevity of organ. In this study, we showed that expression of Tert was impaired in OMSCs, and inhibition of age-induced miR-195 reactivated Tert expression in OMSCs, thereby lengthening telomeres and improving regenerative functions of the aged stem cells. There are many other target genes of miR-195. However, we found that Tert is highly downregulated in aging stem cells which is one of the putative target of miR-195. Since telomerase deactivation with aging is a most commonly accepted process for cellular senescence, we reasoned Tert as a major target of miR-195 for stem cell aging. However, we do not rule out the possibility that miR-195 targets other genes for aging. As an additional mechanism, Bcl-2, an antiapoptotic gene, was also found to be a target of miR-195, and our preliminary data revealed that knockdown of miR-195 increased Bcl-2 expression, thereby reducing apoptosis of stem cells upon H2O2 stimulation (unpublished data). It also remains to be investigated whether overexpression of miR-195 in other stem cell types such as HSCs, CSC, or other tissue-resident stem cells impairs their function. It is also undetermined whether miR-195 expression is induced in human stem cell types and inhibits Tert expression in human cells. Furthermore, the role for the other miRNAs found in the microarray-based profiling in this study (miR-29b, miR-205, miR-378 and miR-542-3p; downregulated with aging, Fig. 2C), is not known. Although our data indicated that abrogation of age-induced miR-195 alone can partially reverse stem cell aging and restore normal function, it is possible that combination with the other age-regulated miRNAs (upregulated or downregulated with aging) may contribute to full restoration of stem cell functions. We have found that at least three miRNAs, including miR-140, miR-146a/b, and miR-195, were significantly upregulated in OMSCs. The combination of miR-195 inhibition and involvement of other miRNAs may have a synergistic effect on rejuvenation of stem cells as well as improvement of heart function in aged hearts.
Further in depth studies are needed to understand the mechanistic participation of age-regulated miRNAs in age related abnormalities of stem cells. We also observed that the effects of YMSCs and modified OMSCs were similar on cardiac repair (data not shown). Infarction size was not significantly different between the use of YMSCs and modified OMSCs. Furthermore, we previously reported that proliferation and differentiation abilities of modified OMSCs were also improved to the level of YMSCs [13]. However, additional studies are required to further understand rejuvenation of stem cells and its impact on repair of aging heart.
Conclusions
This study demonstrated that age-induced miR-195 is one of the key regulators of bone marrow-derived stem cell aging by targeting telomerase. The abrogation of miR-195 is a novel promising strategy to reverse aging clock in the senescent stem cells for repairing ischemic myocardium in elderly patients.
Acknowledgments
This work was supported by National Heart, Lung and Blood Institute grants R37-HL-074272,HL-095375, and HL-087246 (M. Ashraf). National Heart, Lung and Blood Institute #R37-HL-074272 #HL-095375; #HL-087246.
Author Contributions
M.O. and H.W.K.: experimental design, performed experiments, and collection and analysis of data; M.S.: writing and final approval of manuscript; K.M.: performed experiments and collection of data; Y.G.W. and M.X.: interpretation of data and manuscript writing and final approval of manuscript; M.A.: conception and design, financial support, manuscript writing, and final approval of manuscript. M.O. and H.W.K. contributed equally to this work.
Disclosure of Potential Conflicts of Interest
The authors indicate no potential conflicts of interest. This work was carried out at the University of Cincinnati; data were analyzed and finalized there. The manuscript was submitted by the senior author after he joined the University of Illinois at Chicago.
References
1. Körbling M, Estrov Z. Adult stem cells for tissue repair—A new therapeutic concept? N Engl J Med 2003;349:570–58.
CrossRef,PubMed,Web of Science® Times Cited: 420
2. Jones DL, Rando TA. Emerging models and paradigms for stem cell aging. Nat Cell Biol 2011;13: 506–512.
CrossRef,PubMed,CAS,Web of Science® Times Cited: 76
3. von Zglinicki T, Martin-Ruiz CM. Telomeres as biomarkers for ageing and age-related diseases. Curr Mol Med 2005;5:197–203.
CrossRef,PubMed,Web of Science® Times Cited: 139
4. Gavory G, Farrow M, Balasubramanian S. Minimum length requirement of the alignment domain of human telomerase RNA to sustain catalytic activity in vitro. Nucleic Acids Res 2002;30:4470–80.
CrossRef,PubMed,Web of Science® Times Cited: 20
5. Blasco MA, Lee HW, Hande MP et al. Telomere shortening and tumor formation by mouse cells lacking telomerase RNA. Cell 1997;91:25–34.
CrossRef,PubMed,CAS,Web of Science® Times Cited: 1367
6. Sahin E, DePinho RA. Linking functional decline of telomeres, mitochondria and stem cells during ageing. Nature 2010;464:520–528.
CrossRef,PubMed,CAS,Web of Science® Times Cited: 241,ADS
7. Leri A, Franco S, Zacheo A et al. Ablation of telomerase and telomere loss leads to cardiac dilatation and heart failure associated with p53 upregulation. EMBO J 2003;22:131–139.
Direct Link:
AbstractFull Article (HTML)PDF(268K)ReferencesWeb of Science® Times Cited: 138
8. Wong LS, de Boer RA, Samani NJ et al. Telomere biology in heart failure. Eur J Heart Failure 2008;10:1049–1056.
Direct Link:
AbstractFull Article (HTML)PDF(411K)ReferencesWeb of Science® Times Cited: 26
9. Oh H, Wang SC, Prahash A et al. Telomere attrition and Chk2 activation in human heart failure. Proc Natl Acad Sci USA 2003;100:5378–5383.
CrossRef,PubMed,CAS,Web of Science® Times Cited: 98,ADS
10. Chimenti C, Kajstura J, Torella D et al. Senescence and death of primitive cells and myocytes lead to premature cardiac aging and heart failure. Circ Res 2003;93:604–613.
CrossRef,PubMed,CAS,Web of Science® Times Cited: 202
11. Stolzing A, Jones E, McGonagle D et al. Age-related changes in human bone marrow-derived mesenchymal stem cells: Consequence for cell therapies. Mech Ageing Dev 2008;129:163–173.
CrossRef,PubMed,CAS,Web of Science® Times Cited: 338
12. Kucia M, Reca R, Campbell FR et al. A population of very small embryonic-like (VSEL) CXCR4(+)SSEA-1(+)Oct-4 + stem cells identified in adult bone marrow. Leukemia 2006;20:857–869.
CrossRef,PubMed,CAS,Web of Science® Times Cited: 377
13. Igura K, Okada M, Kim HW et al. Identification of small juvenile stem cells in aged bone marrow and their therapeutic potential for repair of the ischemic heart. Am J Physiol Heart Circ Physiol 2013;305:H1354–H1362.
CrossRef,PubMed,CAS,Web of Science® Times Cited: 1
14. Faraonio R, Pane F, Intrieri M et al. In vitro acquired cellular senescence and aging-specific phenotype can be distinguished on the basis of specific mRNA expression. Cell Death Differ 2002;9:862–864.
CrossRef,PubMed,Web of Science® Times Cited: 8
15. Hackl M, Brunner S, Fortschegger K et al. miR-17, miR-19b, miR-20a and miR-106a are downregulated in human aging. Aging Cell 2010;9:291–296.
Direct Link:
AbstractFull Article (HTML)PDF(215K)ReferencesWeb of Science® Times Cited: 127
16. Faraonio R, Salerno P, Passaro F et al. A set of miRNAs participates in the cellular senescence program in human diploid fibroblasts. Cell Death Differ 2012;19:713–721.
CrossRef,PubMed,CAS,Web of Science® Times Cited: 41
17. Poon SS, Martens UM, Ward RK et al. Telomere length measurements using digital fluorescence microscopy. Cytometry 1999;36:267–278.
Direct Link:
AbstractPDF(647K)ReferencesWeb of Science® Times Cited: 129
18. Kim HW, Jiang S, Ashraf M et al. Stem cell-based delivery of Hypoxamir-210 to the infarcted heart: Implications on stem cell survival and preservation of infarcted heart function. J Mol Med 2012;90:997–1010.
CrossRef,PubMed,CAS,Web of Science® Times Cited: 10
19. Kim HW, Haider HK, Jiang S et al. Ischemic preconditioning augments survival of stem cells via miR-210 expression by targeting caspase-8-associated protein 2. J Biol Chem 2009;284:33161–33168.
CrossRef,PubMed,CAS,Web of Science® Times Cited: 158
20. Kim SW, Kim HW, Huang W et al. Cardiac stem cells with electrical stimulation improve ischemic heart function through regulation of connective tissue growth factor and miR-378. Cardiovasc Res 2013;100:241–251.
CrossRef,PubMed,CAS,Web of Science® Times Cited: 6
21. Maggioni AA, Maseri A, Fresco C et al. Age-related increase in mortality among patients with first myocardial infarctions treated with thrombolysis. N Engl J Med 1993;329:1442–1448.
CrossRef,PubMed,CAS,Web of Science® Times Cited: 279
22. Weissman IL. Stem cells: Units of development, units of regeneration, and units in evolution. Cell 2000;100:157–168.
CrossRef,PubMed,CAS,Web of Science® Times Cited: 899
23. Sharpless NE, DePinho RA. How stem cells age and why this makes us grow old. Nat Rev Mol Cell Biol 2007;8:703–713.
CrossRef,PubMed,CAS,Web of Science® Times Cited: 375
24. Dorshkind K, Montecino-Rodriguez E, Signer RA. The ageing immune system: Is it ever too old to become young again? Nat Rev Immunol 2009;9:57–62.
CrossRef,PubMed,CAS,Web of Science® Times Cited: 144
25. Wang Y, Scheiber MN, Neumann C et al. MicroRNA regulation of ionizing radiation-induced premature senescence. Int J Radiat Oncol Biol Phys 2011;81:839–848.
CrossRef,PubMed,CAS,Web of Science® Times Cited: 20
26. Boon RA, Iekushi K, Lechner S et al. MicroRNA-34a regulates cardiac ageing and function. Nature 2013;495:107–110.
CrossRef,PubMed,CAS,Web of Science® Times Cited: 128,ADS
27. Hiyama E, Hiyama K. Telomere and telomerase in stem cells. Br J Cancer 2007;96:1020–1024.
CrossRef,PubMed,CAS,Web of Science® Times Cited: 152
28. Linton PJ, Dorshkind K, Age-related changes in lymphocyte development and function. Nat Immunol 2004:5:133–139.
CrossRef,PubMed,CAS,Web of Science® Times Cited: 511
29. Miller RA. The aging immune system: Primer and prospectus. Science 1996;273:70–74.
CrossRef,PubMed,CAS,Web of Science® Times Cited: 746,ADS
30. Globerson A, Effros RB. Ageing of lymphocytes and lymphocytes in the aged. Immunol Today 2000;21:515–521.
CrossRef,PubMed,CAS,Web of Science® Times Cited: 194
31. Stenderup K, Justesen J, Clausen C et al. Aging is associated with decreased maximal life span and accelerated senescence of bone marrow stromal cells. Bone 2003;33:919–926.
CrossRef,PubMed,Web of Science® Times Cited: 539
32. Simonsen JL, Rosada C, Serakinci N et al. Telomerase expression extends the proliferative life-span and maintains the osteogenic potential of human bone marrow stromal cells. Nat Biotechnol 2002;20:592–596.
CrossRef,PubMed,CAS,Web of Science® Times Cited: 409
33. Liu L, DiGirolamo CM, Navarro PA et al. Telomerase deficiency impairs differentiation of mesenchymal stem cells. Exp Cell Res 2004;294:1–8.
CrossRef,PubMed,CAS,Web of Science® Times Cited: 65